EIDD-2801 works similarly to Gilead Sciences’ remdesivir, an unapproved drug that was developed for the Ebola virus and is being studied in five Phase III trials against COVID-19. Both molecules are nucleoside analogs that metabolize into an active form that blocks RNA polymerase, an essential component of viral replication.
Home » Posts tagged 'COVID-19'
Tag Archives: COVID-19
ARCT-021 (LUNAR-COV19)
ARCT-021 (LUNAR-COV19)
cas 2541451-24-3
A lipid-enabled and UnlockedNucleomonomer Agent modified RNA (LUNAR) of self-replicating RNA for vaccination against spike protein of SARS-CoV-2 (Arcturus)
Self-replicating RNA vaccine
Arcturus Therapeutics and Duke-NUS Medical School, Singapore |
- OriginatorArcturus Therapeutics
- ClassCOVID-19 vaccines; RNA vaccines; Viral vaccines
- Mechanism of ActionImmunostimulants
- Orphan Drug StatusNo
- New Molecular EntityNo
- Available For LicensingYes – COVID 2019 infections
- Phase IICOVID 2019 infections
- 01 Mar 2021Arcturus Therapeutics has patent pending for STARR platform in USA
- 01 Mar 2021Immunogenicity data from a preclinical studies in COVID-2019 infections released by Arcturus Therapeutics
- 01 Mar 2021Arcturus Therapeutics completes a phase I/II trial in COVID-2019 infection in the Singapore
ref International Journal of Biological Sciences (2021), 17(6), 1446-1460. https://www.ijbs.com/v17p1446.htm
LUNAR-COV19 | T7 | m7GpppNmN | Yes | VEEV-FL-S | N1-methyl pseudouridine | Silicon column | |
protein | [54] |
ARCT-021: Currently undergoing phase 1/2 clinical trials, it combines two technologies, i.e., saRNA STARR™ and LUNAR® lipid-mediated delivery method. It was designed to enhance and extend antigen expression, enabling vaccination at lower doses [87]. In addition, LUNAR® lipids are pH-sensitive and biodegradable, causing minimal lipid accumulation in cells after multiple dosing [87]The Arcturus COVID-19 vaccine, commonly known as ARCT-021 and LUNAR-COV19, is a COVID-19 vaccine candidate developed by Arcturus Therapeutics.
LUNAR- COV19 | 1 | Day 0 | 0.2 μg and 10 μg (Preclinical) | IM | Arcturus Therapeutics | N/A | Phase 2 | NCT04668339 NCT04480957 | [54] |
54. de Alwis R, Gan ES, Chen S, Leong YS, Tan HC, Zhang SL. et al. A Single Dose of Self-Transcribing and Replicating RNA Based SARS-CoV-2 Vaccine Produces Protective Adaptive Immunity In Mice. bioRxiv. 2020. 2020 09.03.280446
Development
Arcturus Therapeutics partnered with Singapore’s Duke–NUS Medical School to develop a COVID-19 vaccine.[1] The company also partnered with Catalent, a contract development and manufacturing organization, to manufacture multiple batches of Arcturus’ COVID-19 mRNA vaccine candidate.[2]
Clinical research
Phase I-II
LUNAR-COV19 clinical trials in humans began in July 2020.[3] On 4 January 2021, Arcturus Therapeutics started phase-2 clinical trials.[4]
Deployment
Arcturus has entered into development and supply agreements with the Economic Development Board of Singapore and supply agreements with the Israel Ministry of Health for LUNAR-COV19.[5][6]
Arcturus Therapeutics Receives FDA Allowance to Proceed with Phase 2 Study of ARCT-021 (LUNAR-COV19) Vaccine Candidate in the United States
Phase 2 study to be conducted in the U.S. and Singapore, and will evaluate both single dose and two dose priming regimens of ARCT-021 in up to 600 participants
Anticipate interim Phase 2 data in early 2021; targeting global Phase 3 study start in Q2 2021 which could allow application for emergency use authorization/conditional approval in H2 2021January 04, 2021 07:01 AM Eastern Standard Time
SAN DIEGO–(BUSINESS WIRE)–Arcturus Therapeutics Holdings Inc. (the “Company”, “Arcturus”, Nasdaq: ARCT), a leading clinical-stage messenger RNA medicines company focused on the development of infectious disease vaccines and significant opportunities within liver and respiratory rare diseases, today announced that the Company has received allowance of the Investigational New Drug (IND) application from the U.S. Food and Drug Administration (FDA) for the Phase 2 clinical study of its vaccine candidate ARCT-021 following review of data from the Phase 1/2 study.
Arcturus Therapeutics Receives FDA Allowance to Proceed with Phase 2 Study of ARCT-021 (LUNAR-COV19) Vaccine Candidate in the United States
Arcturus previously announced that the ARCT-021 Phase 2 study had been approved to proceed by the Singapore Health Sciences Authority (HSA), who reviewed the same data as reviewed by the FDA. These Phase 1/2 study results demonstrated favorable tolerability and both humoral and cellular immunogenicity following administration of ARCT-021.
The Phase 2 study will enroll 600 participants, with 450 receiving ARCT-021 and 150 receiving placebo. Both older and younger adult participants will be included. Early interim analyses of safety and immunogenicity will be performed to inform dose selection for a Phase 3 study, which is targeted to start in Q2 2021, if the Phase 2 study is successful.
“Allowance of the IND for our ARCT-021 Phase 2 clinical study represents an important milestone for the program and we look forward to starting to screen study participants at U.S. and Singapore clinical sites very soon,” said Steve Hughes, M.D., Chief Medical Officer of Arcturus. “We have advanced ARCT-021 to Phase 2 based on promising interim results from our Phase 1/2 study and extensive preclinical data. Our prior clinical results show that ARCT-021 administration results in humoral and cellular immunogenicity, and we are encouraged by an increasing body of evidence highlighting the potential importance of T cells in providing protection against SARS-CoV-2 infection and COVID-19. We believe that ARCT-021 holds promise to be a highly effective vaccine with a differentiated clinical profile, including the potential to only require a single dose for protection.”
About Arcturus Therapeutics
Founded in 2013 and based in San Diego, California, Arcturus Therapeutics Holdings Inc. (Nasdaq: ARCT) is a clinical-stage mRNA medicines and vaccines company with enabling technologies: (i) LUNAR® lipid-mediated delivery, (ii) STARR™ mRNA Technology and (iii) mRNA drug substance along with drug product manufacturing expertise. Arcturus’ diverse pipeline of RNA therapeutic and vaccine candidates includes self-replicating mRNA vaccine programs for SARS-CoV-2 (COVID-19) and Influenza, and other programs to potentially treat Ornithine Transcarbamylase (OTC) Deficiency, Cystic Fibrosis, and Cardiovascular Disease along with partnered programs including Glycogen Storage Disease Type 3, Hepatitis B Virus, and non-alcoholic steatohepatitis (NASH). Arcturus’ versatile RNA therapeutics platforms can be applied toward multiple types of nucleic acid medicines including messenger RNA, small interfering RNA, replicon RNA, antisense RNA, microRNA, DNA, and gene editing therapeutics. Arcturus’ technologies are covered by its extensive patent portfolio (205 patents and patent applications, issued in the U.S., Europe, Japan, China and other countries). Arcturus’ commitment to the development of novel RNA therapeutics has led to collaborations with Janssen Pharmaceuticals, Inc., part of the Janssen Pharmaceutical Companies of Johnson & Johnson, Ultragenyx Pharmaceutical, Inc., Takeda Pharmaceutical Company Limited, CureVac AG, Synthetic Genomics Inc., Duke-NUS Medical School, and the Cystic Fibrosis Foundation. For more information visit www.ArcturusRx.com. In addition, please connect with us on Twitter and LinkedIn.
References
- ^ Teo J (15 April 2020). “Coronavirus: Clinical trials for Singapore’s vaccine project could start in August”. The Straits Times. Retrieved 27 April 2020.
- ^ Stanton D (6 May 2020). “With Arcturus, Catalent bags another COVID project”. Bioprocess Insider. Retrieved 8 May 2020.
- ^ Clinical trial number NCT04480957 for “Phase 1/2 Ascending Dose Study of Investigational SARS-CoV-2 Vaccine ARCT-021 in Healthy Adult Subjects” at ClinicalTrials.gov
- ^ “Arcturus Therapeutics Receives FDA Allowance to Proceed with Phase 2 Study of ARCT-021 (LUNAR-COV19) Vaccine Candidate in the”. Bloomberg. 4 January 2021. Retrieved 17 January 2021.
- ^ Anwar N (26 November 2020). “Singapore’s co-developed vaccine candidate is in ‘good shape’ for delivery in 2021”. CNBC. Retrieved 18 March 2021.
- ^ Cheok M, Mookerjee I (5 August 2020). “Singapore Will Get First Claim to Any Successful Arcturus Vaccine”. Bloomberg. Retrieved 18 March 2021.
External links
![]() | Scholia has a profile for Lunar-COV19 (Q98713328). |
Vaccine description | |
---|---|
Target | SARS-CoV-2 |
Vaccine type | mRNA |
Clinical data | |
Other names | ARCT-021, LUNAR-COV19 |
Routes of administration | Intramuscular |
Part of a series on the |
COVID-19 pandemic |
---|
COVID-19 (disease)SARS-CoV-2 (virus) |
showTimeline |
showLocations |
showInternational response |
showMedical response |
showImpact |
COVID-19 portal |
/////////COVID-19, SARS-CoV-2, corona virus, singapore, ARCT 021, LUNAR-COV19

NEW DRUG APPROVALS
one time
$10.00
IIBR-100
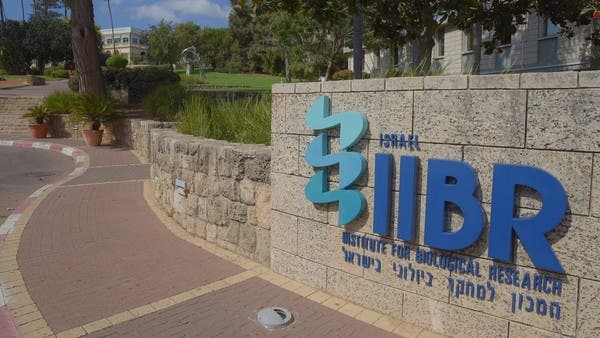
IIBR-100
Brilife
Recombinant vesicular stomatitis virus (rVSV) vaccine
Israel Institute for Biological Research
Hadassah Medical Center; Sheba Medical Center Hospital
The SARS-CoV-2 virus is responsible for the COVID-19 pandemic. The pandemic emerged from Wuhan Province in China in December 2019 and was declared by the WHO Director-General a Public Health Emergency of International Concern on 30 January 2020.
In this study, a vaccine developed by IIBR for SARS-CoV-2 virus will be assessed for its safety and potential efficacy in volunteers. The study is comprised of two phases, a dose-escalation phase (phase I) during which subjects (18-55 years old) will be randomly allocated to receive a single administration of IIBR-100 100 at low, mid or high dose or saline or two administrations of IIBR-100 at low dose, or saline, 28 days apart.
Based on results obtained during phase I, and cumulative phase I data review, the expansion phase (phase II) has begun, during which larger cohorts as well as elderly age subjects will be randomly allocated to receive a single administration of IIBR-100 at low, mid or high dose or saline, or two administrations of IIBR-100 at low, mid or high dose (prime-boost) or saline, 28 days apart. Additional top-dose (prime-boost) may be implemented when immunogenicity of any prime-boost arm is considered insufficient.
Based on immunogenicity preliminary data and DSMB recommendations, the two administrations of mid, high and top dose (prime-boost) or saline will continue.
The subjects will be followed for a period of up to 12 months post last vaccine administration to assess the safety and efficacy of the vaccine.
https://clinicaltrials.gov/ct2/show/NCT04608305
IIBR-100 also known as Brilife is a COVID-19 vaccine candidate developed by The Israel Institute for Biological Research.[1][2]
References
- ^ Clinical trial number NCT04608305 for “Phase I/II Randomized, Multi-Center, Placebo-Controlled, Dose-Escalation Study to Evaluate the Safety, Immunogenicity and Potential Efficacy of an rVSV-SARS-CoV-2-S Vaccine (IIBR-100) in Adults” at ClinicalTrials.gov
- ^ Jeffay N (29 December 2020). “As Israel goes vaccine-wild, will the homegrown version lose its shot?”. The Times of Israel. Retrieved 1 January 2021.
candidate developed by The Israel Institute for Biological Research.[1][2]
Israeli institute’s COVID vaccine candidate said very effective in animal trials
Secretive Israeli research center’s shot shows near 100% efficacy in non-human trials, is on par with US company Moderna’s candidate, TV report says
Israeli researchers at a top secret research center have made progress on a coronavirus vaccine that shows a high level of effectiveness in animals, according to a Friday TV report.
However, there is no guarantee that the vaccine under development will be effective in humans, or will be available soon.
The Israel Institute for Biological Research (IIBR), a secretive unit that works under the Prime Minister’s Office, developed a vaccine that shows close to 100 percent protection against the virus in lab animals, the Channel 12 report said, citing “a security source.”
The vaccine under development is on par in effectiveness with a vaccine being developed by US biotechnology company Moderna, the report said.
Unlike vaccines developed abroad, the domestic vaccine will first be delivered to Israeli citizens, it added. If successful, it was expected to provide protection against the disease with a single dose.
The institute has not started human trials but was preparing to manufacture 10 to 15 million doses, report said.
Hebrew media have reported on potential breakthroughs at the shadowy institute several times before, starting in mid-March, with the Defense Ministry pushing back on some of the claims to tamper expectations.

Magen David Adom medical workers test Israelis for the coronavirus at a drive-through site in Lod, on July 10, 2020. (Yossi Aloni/Flash90)
IIBR said last month that it had completed successful coronavirus vaccine trials on rodents, paving the way for further testing on other animals and then possibly human trials.
In a paper published on the website of bioRxiv, an online repository for papers that haven’t yet been peer-reviewed, the institute, which is based in Ness Ziona, said it hopes to have a finished vaccine in a year, or possibly even earlier.
In the abstract of the report, the researchers say their vaccine, which they tested on hamsters, “results in rapid and potent induction of neutralizing antibodies against SARS-CoV-2,” the virus that causes COVID-19.
Earlier this month a vaccine adviser to the government cautioned that there was no guarantee that the shots being developed will prove widely effective.
In May, the institute confirmed that it had isolated an antibody it believed could be used to develop treatments against the virus. The development would not be useful in the creation of a vaccine, but would rather be a move toward a drug treatment for those who have already contracted the disease.
Tal Zaks, Moderna’s Israeli chief medical officer, described to Channel 12 on Friday the company’s push into Phase 3 testing of its vaccine candidate, which was developed with the National Institutes of Health, and began its first injections Monday.
The trial, the world’s largest vaccine study, plans to test the vaccine on 30,000 volunteers.
There’s still no guarantee that the experimental vaccine, developed by the National Institutes of Health and Moderna Inc., will really offer protection.
“The first time we saw the first model, that the vaccine, even if it’s just in mice, successfully stimulated the immune system to identify the virus and neutralize it, I knew that we hadn’t missed anything, that we had the correct vaccine,” he said.
“And of course the second ‘ah-ha’ moment was when we saw the first clinical results, when it was clear that in humans we weren’t just getting to antibody levels we were seeing in sick people, which is what we aspired to, but we were getting to even higher levels,” Zaks said.

A Nurse gives a volunteer an injection, as the world’s biggest study of a possible COVID-19 vaccine, developed by the US National Institutes of Health and Moderna Inc., gets underway on July 27, 2020, in Binghamton, NY. (AP Photo/Hans Pennink)
Last month Israel signed a deal with Moderna for the potential purchase of its coronavirus vaccine if it ends up proving effective.
Moderna said the vaccination was administered in Savannah, Georgia, the first site to get underway among more than seven dozen trial sites scattered around the country.
Several other vaccines made by China and by Britain’s Oxford University earlier this month began smaller final-stage tests in Brazil and other hard-hit countries.
The massive studies aren’t just to test if the shots work — they’re needed to check each potential vaccine’s safety. And following the same study rules will let scientists eventually compare all the shots.
It normally takes years to create a new vaccine from scratch, but scientists are setting speed records this time around, spurred by knowledge that vaccination is the world’s best hope against the pandemic.
If everything goes right with the final studies, it still will take months for the first data to trickle in from the Moderna test, followed by the Oxford one.
Governments around the world are trying to stockpile millions of doses of those leading candidates so if and when regulators approve one or more vaccines, immunizations can begin immediately. But the first available doses will be rationed, presumably reserved for people at highest risk from the virus.
Coronavirus cases in Israel rose by 1,791 in 24 hours on Friday and the national death toll hit 512, according to the latest Health Ministry figures.
The total case count stood at 70,970, with 320 patients in serious condition, including 98 on ventilators. The number of recovered patients reached 43,850.
Israel has the fifth-highest number of new coronavirus infections per capita in the world, overtaking the United States, according to data compiled by a scientific publication based at Oxford University.
And while Israel has seen the number of new coronavirus cases rocket to more than 2,000 a day in recent weeks, a new Hebrew University report published on Thursday asserted that Israel has managed to gain control of the second wave of the coronavirus, thanks to a recent stabilization in the number of seriously and moderately ill patients.
The curve for seriously and moderately ill patients began to spike in late June before stabilizing in recent days, the researchers reported. They credited the restrictions imposed by the government in recent weeks to limit crowding for helping to flatten the curve.
According to the report, the death toll will climb by roughly 200 in the coming three weeks as a result of the high infection rate over the past month.
Experts have blamed a too-speedy reopening and the lack of an effective contact-tracing program as main factors in the virus resurgence, which has come as new daily coronavirus cases around the world have also reached record highs.
Vaccine description | |
---|---|
Target | SARS-CoV-2 |
Vaccine type | Viral vector |
Clinical data | |
Other names | Brilife |
Routes of administration | Intramuscular |
Part of a series on the |
COVID-19 pandemic |
---|
COVID-19 (disease)SARS-CoV-2 virus (variants) |
showTimeline |
showLocations |
showInternational response |
showMedical response |
showImpact |
COVID-19 portal |
//////IIBR-100, Brilife, COVID-19, vaccine, israel, corona virus, covid 19, SARS-CoV-2
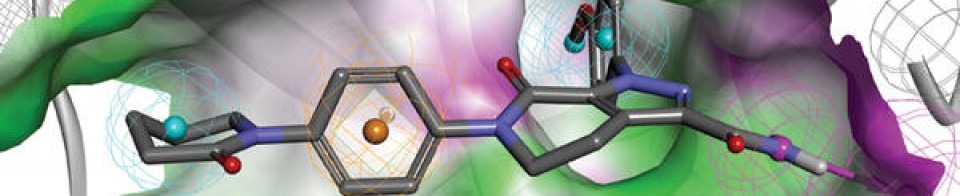
NEW DRUG APPROVALS
one time
$10.00
ABDALA, CIGB-66

ABDALA
CIGB-66, is a COVID-19 vaccine
Cuba says Abdala vaccine 92.28% effective against coronavirus
The announcement came just days after the government said another homegrown vaccine, Soberana 2, has proved to be 62% effective with just two of its three doses.
- June 22, 2021, 10:03 IST
Havana: Cuba said on Monday that its three-shot Abdala vaccine against the coronavirus has been proved 92.28% effective in last-stage clinical trials.
The announcement came just days after the government said another homegrown vaccine, Soberana 2, has proved to be 62% effective with just two of its three doses.
“Hit by the pandemic, our scientists at the Finlay Institute and Center for Genetic Engineering and Biotechnology have risen above all the obstacles and given us two very effective vaccines,” President Miguel Diaz-Canel tweeted.
The announcement came from state-run biopharmaceutical corporation BioCubaFarma, which oversees Finlay, the maker of Soberana 2, and the Center for Genetic Engineering and Biotechnology, the producer of Abdala.
Both vaccines are expected to be granted emergency authority by local regulators shortly.
Cuba, whose biotech sector has exported vaccines for decades, has five coronavirus vaccine candidates.
The Caribbean’s largest island is facing its worst Covid-19 outbreak since the start of the pandemic following the arrival of more contagious variants, setting new records for daily coronavirus cases.
The Communist-run country has opted not to import foreign vaccines but to rely on its own. Some experts said it was a risky bet but it appears to have paid off, putting Cuba in position to burnish its scientific reputation, generate much-needed hard currency through exports and strengthen the vaccination drive worldwide.
Several countries from Argentina and Jamaica to Mexico, Vietnam and Venezuela have expressed an interest in buying Cuba’s vaccines. Iran started producing Soberana 2 earlier this year as part of late-phase clinical trials.
Cuba’s authorities have already started administering the experimental vaccines en masse as part of “intervention studies” they hope will slow the spread of the virus.
About a million of the country’s 11.2 million residents have been fully vaccinated to date.
Daily cases have halved in the capital, Havana, since the start of the vaccination campaign a month ago, using Abdala, according to official data.
Cuba has reported a total of 169,365 Covid-19 cases and 1,170 deaths.
ABDALA, technical name CIGB-66, is a COVID-19 vaccine candidate developed by the Center for Genetic Engineering and Biotechnology in Cuba.[1][2] This vaccine candidate, named after a patriotic drama by Cuban independence hero José Martí, is a protein subunit vaccine containing COVID-derived proteins that trigger an immune response.[3] However, none of the clinical trial full results have been published. This candidate followed a previous one called CIGB-669 (MAMBISA).[4]
The vaccine is one of two Cuba-developed COVID-19 vaccines in Phase III trials.[5][6][7]
Clinical research
Phase I/II
In July 2020, CIGB-66 commenced phase I/II clinical trials.[8]
Phase III
The Phase III trial compares 3 doses of the vaccine administered at 0, 14 and 28 days against a placebo, with the primary outcome measuring the proportion of cases reported for each group 14 days after the third dose.
The trial was registered on 18 March 2021. The first dose was administered on 22 March and by April 4, the 48,000 participants had received their first dose,[9][10] and second doses started being administered from April 5.[11][12] Third doses have started being administered on 19 April[13][14][15] and on May 1, 97% of the original participants had received their 3 doses, the others 3% were lost in the process.
Intervention study
124,000 people aged 19 to 80 received 3 doses of the vaccine as part of an intervention study, with the primary outcome measuring the proportion of cases and deaths for the vaccinated compared to the unvaccinated population.[16]
A wider intervention study with the 1.7 million inhabitants of Havana is expected to start in May with the ABDALA and Soberana 2 vaccine.[17]
Efficacy
From May 3, the efficacy of the vaccine will start being evaluated.[18][19][20]
The “first evaluation of efficacy” can begin when there is 50 cases, then there is a second evaluation at 100 cases and a definitive efficacy can “finally be demonstrated” at 150 cases, Cuban Center for Genetic Engineering and Biotechnology director said.[21]
Production outside Cuba
Venezuela has claimed that it will manufacture the vaccine[22] but this claim has not yet materialised.[23] State-owned EspromedBIO will manufacture the vaccine but some “arrangements” are needed to start production.[24] In April, Nicolás Maduro said that a capacity of 2 Million doses per month is hoped to be reach by “August, September approximately”.[25
In June 2021, Vietnam’s Ministry of Health announced that negotiations were ongoing between Cuba and Vietnam for Abdala vaccine production. The Institute of Vaccines and Medical Biologicals (IVAC) was named as the focal point for receiving technology transfer.[26]
References
- ^ “ABDALA Clinical Study – Phase III”. rpcec.sld.cu. Registro Público Cubano de Ensayos Clínicos. Retrieved 22 March 2021.
- ^ “ABDALA Clinical Study”. rpcec.sld.cu. Registro Público Cubano de Ensayos Clínicos. Retrieved 22 March 2021.
- ^ Yaffe H (31 March 2021). “Cuba’s five COVID-19 vaccines: the full story on Soberana 01/02/Plus, Abdala, and Mambisa”. LSE Latin America and Caribbean blog. Retrieved 31 March 2021.
- ^ “MAMBISA Study”. rpcec.sld.cu. Registro Público Cubano de Ensayos Clínicos. Retrieved 22 March 2021.
- ^ “Three-shot Cuban COVID-19 vaccine candidate moves forward in phase III”. http://www.bioworld.com. Retrieved 10 April 2021.
- ^ “Cuba’s Abdala COVID-19 vaccine enters phase 3 clinical trial – Xinhua | English.news.cn”. http://www.xinhuanet.com. Retrieved 10 April 2021.
- ^ Zimmer C, Corum J, Wee SL. “Coronavirus Vaccine Tracker”. The New York Times. ISSN 0362-4331. Retrieved 10 April 2021.
- ^ “ABDALA Clinical Study”. rpcec.sld.cu. Registro Público Cubano de Ensayos Clínicos. Retrieved 21 March 2021.
- ^ BioCubaFarma (4 April 2021). “[Translated] “The application of the 1st dose of #Abdala, in volunteer 48 thousand, of the Phase III Clinical Trial. Next Monday, April 5, the application of the 2nd dose of this vaccine candidate begins. #VcaunasCubanasCovid19 .””. Twitter. Retrieved 10 April 2021.
- ^ “Covid Check-in: Cuba’s Homegrown Vaccines”. AS/COA. Retrieved 10 April 2021.
- ^ BioCubaFarma (5 April 2021). “[Translated] “The application of the 2nd dose of the vaccine candidates begins today #Abdala and #Soberana02 , as part of the 3rd phase of the clinical trial. Workers of @Emcomed1 in Havana and eastern provinces, from very early hours they carry out their distribution until the vaccination centers””. Twitter (in Spanish). Retrieved 10 April 2021.
- ^ “Two Cuban Vaccines Start Second Dose Phase III Trials”. Kawsachun News. 5 April 2021. Retrieved 10 April 2021.
- ^ “Abdala: Comienza tercera dosis en el Oriente cubano”. http://www.cuba.cu (in Spanish). Retrieved 21 April 2021.
- ^ BioCubaFarma. “[Translated] “Application of the 3rd dose of the vaccine candidate begins #Abdala in the provinces of Granma, Santiago de Cuba and Guantánamo. The application of the 2nd dose of #Soberana02 within the framework of the EC Phase III.#VacunasCubanasCovid19”. Twitter. Retrieved 21 April 2021.
- ^ Noticias, Agencia Cubana de. “Convergen múltiples voluntades para éxito de estudio Abdala en Bayamo”. ACN (in Spanish). Retrieved 21 April 2021.
- ^ “ABDALA-Intervention | Registro Público Cubano de Ensayos Clínicos”. rpcec.sld.cu. Retrieved 10 April 2021.
- ^ Ministerio de Salud Pública en Cuba. “Sitio oficial de gobierno del Ministerio de Salud Pública en Cuba”. Sitio oficial de gobierno del Ministerio de Salud Pública en Cuba (in Spanish). Retrieved 23 April 2021.
- ^ “Scientists announce Abdala’s administration of 3rd dose will finish”. http://www.plenglish.com/index.php?o=rn&id=66941&SEO=scientists-announce-abdalas-administration-of-3rd-dose-will-finish (in Spanish). Retrieved 2 May 2021.
- ^ Noticias, Agencia Cubana de. “Concluye aplicación de vacuna Abdala en Oriente de Cuba”. ACN (in Spanish). Retrieved 2 May2021.
- ^ “Cuba conclui ensaios clínicos de candidata a vacina contra covid-19”. R7.com (in Portuguese). 2 May 2021. Retrieved 2 May2021.
- ^ “Abdala cerca de concluir la fase III de ensayos clínicos; Mambisa se alista para avanzar a nueva fase (+Video)”. Granma.cu (in Spanish). Retrieved 3 May 2021.
- ^ “Cuba says it’s ‘betting it safe’ with its own Covid vaccine”. NBC News. Retrieved 10 April 2021.
- ^ “Maduro struggles to make his grand vaccine promise”. Eminetra.co.uk. 2 May 2021. Retrieved 3 May 2021.
- ^ “Venezuela producirá la vacuna cubana anticovid Abdala”. http://www.efe.com (in Spanish). Retrieved 3 May 2021.
- ^ Apr 11, Reuters /; 2021; Ist, 16:27. “Indonesian President orders Java rescue efforts after quake kills 8 – Times of India”. The Times of India. Retrieved 3 May 2021.
- ^ Ministry of Health Vietnam (16 June 2021). “Bộ trưởng Bộ Y tế đàm phán với Cuba về hợp tác sản xuất vaccine”. giadinh.net.vn(in Vietnamese). Retrieved 17 June 2021.
External links
![]() | Scholia has a profile for Abdala (Q106390652). |
Vaccine description | |
---|---|
Target | SARS-CoV-2 |
Vaccine type | Protein subunit |
Clinical data | |
Other names | ABDALA |
Routes of administration | Intramuscular |
Part of a series on the |
COVID-19 pandemic |
---|
COVID-19 (disease)SARS-CoV-2 virus (variants) |
showTimeline |
showLocations |
showInternational response |
showMedical response |
showImpact |
COVID-19 portal |
//ABDALA, CUBA, CIGB-66, COVID-19, vaccine, CORONA VIRUS, SARS-CoV-2,
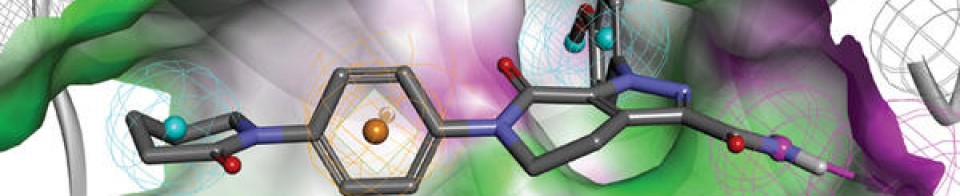
NEW DRUG APPROVALS
ONE TIME
$10.00
PTX-COVID19-B
PTX-COVID19-B
mRNA-based vaccine |
Providence Therapeutics; Canadian government
bioRxiv (2021), 1-50.
https://www.biorxiv.org/content/10.1101/2021.05.11.443286v1
Safe and effective vaccines are needed to end the COVID-19 pandemic caused by SARS-CoV-2. Here we report the preclinical development of a lipid nanoparticle (LNP) formulated SARS-CoV-2 mRNA vaccine, PTX-COVID19-B. PTX-COVID19-B was chosen among three candidates after the initial mouse vaccination results showed that it elicited the strongest neutralizing antibody response against SARS-CoV-2. Further tests in mice and hamsters indicated that PTX-COVID19-B induced robust humoral and cellular immune responses and completely protected the vaccinated animals from SARS-CoV-2 infection in the lung. Studies in hamsters also showed that PTX-COVID19-B protected the upper respiratory tract from SARS-CoV-2 infection. Mouse immune sera elicited by PTX-COVID19-B vaccination were able to neutralize SARS-CoV-2 variants of concern (VOCs), including the B.1.1.7, B.1.351 and P.1 lineages. No adverse effects were induced by PTX-COVID19-B in both mice and hamsters. These preclinical results indicate that PTX-COVID19-B is safe and effective. Based on these results, PTX-COVID19-B was authorized by Health Canada to enter clinical trials in December 2020 with a phase 1 clinical trial ongoing (ClinicalTrials.gov number: NCT04765436).
PTX-COVID19-B is a messenger RNA (mRNA)-based COVID-19 vaccine, a vaccine for the prevention of the COVID-19 disease caused by an infection of the SARS-CoV-2 coronavirus, created by Providence Therapeutics—a private Canadian drug company co-founded by Calgary, Alberta-based businessman Brad T. Sorenson and San Francisco-based Eric Marcusson.[1] in 2013. A team of eighteen working out of Sunnybrook Research Institute in Toronto, Ontario developed PTX-COVID19-B[2] in less than four weeks, according to the Calgary Herald.[3] Human trials with sixty volunteers began on January 26, 2021 in Toronto.[4][5][6]
Providence, which has no manufacturing facilities, partnered with Calgary-based Northern mRNA—the “anchor tenant” in their future manufacturing facilities pending financing.[2]
On 30 April 2021, Sorenson announced that Providence Therapeutics would be leaving Canada and any vaccine that it developed would not be manufactured in Canada.[2]
Overview
Providence Therapeutics Holdings Inc. was co-founded in Toronto, Ontario[7][8] by Calgary, Alberta-based businessman Brad T. Sorenson and San Francisco-based Eric Marcusson Ph.D, who was also the Chief Scientific Officer.[9][3]
PTX-COVID19-B is a messenger RNA (mRNA)-based COVID-19 vaccine. In an interview with CTV news, Sorenson said they were “building some of the important building blocks for the messenger RNA … that provides instructions to cells … to build proteins that may treat or prevent disease”.
As of January 2021, Northern RNA’s Calgary lab was proposed as the site where manufacturing of PTX-COVID19-B would take place.[10] Providence Therapeutics’ partner, Northern RNA, which located at 421 7 Avenue SW in Calgary, has been described as Providence Therapeutics northern division.[7][8]
A February 2021 Manitoba government press release said that the Winnipeg-based Emergent BioSolutions would be manufacturing the vaccine.[11]
Human trials
Phase 1
Human trials began on January 26, 2021 with 60 volunteers between the ages of 18 to 65 in Toronto.[12][13][3] Of these, 15 would receive a placebo and 3 groups of 15 would receive different doses of the vaccine.[10] The volunteers will be monitored for 13 months. The company said that enough data would be available in May which could result in a Phase 2 clinical testing beginning soon after that, pending regulatory approval. If the results of a subsequent larger human trial are positive, the vaccine could enter a commercialization phase in 2022.[14] The Phase 1 clinical trial lead was Piyush Patel. At the 29 April meeting with the House of Commons, Sorenson estimated that PTX-COVID19-B could be approved by Health Canada by “January or February 2022”.[15]:8
Provincial funding
Shortly after the first human trials on PTX-COVID19-B began in late January, on 11 February 2021, Manitoba Premier Brian Pallister announced a “term sheet” between the province and Providence Therapeutics through which Manitoba would receive 2 million doses of PTX-COVID19-B pending its approval by Health Canada.[11] The term sheet includes “best-price guarantee” PTX-COVID19-B.[13] According to a provincial statement released by the Manitoba government, pending approval of the vaccine, the actual manufacturing would take place in Winnipeg by Emergent BioSolutions.[11] Pallister said that, “Building a secure, made-in-Canada vaccine supply will put Canadians at the head of the line to get a COVID vaccine, where we belong.”[11] The down payment would be 20% with a subsequent 40% to be paid when the vaccine was approved by Health Canada; the balance would be paid on delivery of the doses.[13] Specifics about the contract were released in April 2021: the total cost was estimated as CAD $36 million and the agreement included a clause for a non-refundable advance payment of CAD $7.2 million.[2] Sorenson made this comment to Global News: “Under no circumstances is Manitoba going to be on the hook for $7.2 million unless they get real value out of it”.
Federal funding
Canada’s National Research Council (NRC) provided Providence Therapeutics with CAD $5 million for the launch of January 2021 first phase of PTX-COVID19-B clinical trials.[2]
As part of the federal government’s “next generation manufacturing supercluster” program, Providence and Northern mRNA had also been “cleared to access up to $5 million” towards the manufacturing start up process, according to a federal government spokesperson.[2]
The CBC report in late April 2021 also stated that “it could be eligible for a slice of $113 million in additional funding from the National Research Council of Canada Industrial Research Assistance Program”. The federal government had provided funding to some other companies in Canada that were also working to develop a COVID-19 vaccine.[2]
Sorenson as Providence Therapeutics CEO posted an open letter to Prime Minister Justin Trudeau, in which he requested $CDN 150 million upfront to be used to pay for clinical trial and material costs.[16][9]
On 29 April 2021, Sorenson appeared before the House of Commons standing committee on international trade, to ask the Minister of Procurement, Anita Anand, to consider PTX-COVID19-B as an alternative to Moderna and Pfizer for the “2022 booster vaccines”.[15] Sorenson said that the NRC had approached Providence Therapeutics in 2020 after the company had announced their Phase I trial PTX-COVID19-B. Sorenson told the Standing Committee that, “We’ve had really good dialogue ever since phase I started. That process has gone on. That started probably [in February], as we geared up to conclude our phase I trial and release data. Although the NRC is capped at $10 million, which is certainly not sufficient to carry out phase II and phase III trials, the NRC has, through the bureaucracy, elevated us back up to the strategic innovation fund. That occurred about three weeks ago. We’re now working with the strategic innovation fund.”[15]:7
He later said that no reply had been received from the government.[17]
In a meeting with the federal COVID-19 vaccine task force and Sorenson, task force members expressed concerns that “Providence might not be able to scale up production fast enough”.[2]
Clinical trials
PTX-COVID19-B, an mRNA Humoral Vaccine, is Intended for Prevention of COVID-19 in a General Population. This Study is Designed to Evaluate Safety, Tolerability, and Immunogenicity of PTX-COVID19-B Vaccine in Healthy Seronegative Adults Aged 18-64… https://clinicaltrials.gov/ct2/show/NCT04765436
Hyderabad Drugmaker To Make Canada Firm’s mRNA Covid Vaccine In India.. https://www.ndtv.com/india-news/hyderabad-drugmaker-biological-e-to-make-canada-firms-mrna-covid-vaccine-in-india-2454000
Biological E., will run a clinical trial of Providence’s vaccine in India and seek emergency use approval for it, the company said in a statement
Hyderabad-based Biological E said on Tuesday it has entered into a licensing agreement with Providence Therapeutics Holdings to manufacture the Canadian company’s mRNA COVID-19 vaccine in India.
Biological E., which also has a separate deal to produce about 600 million doses of Johnson & Johnson’s COVID-19 shot annually, will run a clinical trial of Providence’s vaccine in India and seek emergency use approval for it, the company said in a statement.
Providence will sell up to 30 million doses of its mRNA vaccine, PTX-COVID19-B, to Biological E, and will also provide the necessary technology transfer of the shot, with a minimum production capacity of 600 million doses in 2022 and a target capacity of 1 billion doses.
Financial details of the transaction were not disclosed.
India has been struggling with a devastating second wave of the pandemic and has managed to fully vaccinate only about 3% of its population. On Monday, the Serum Institute of India said it will increase production of AstraZeneca’s shot by nearly 40% in June, a step towards bridging the shortfall in the country.
“The mRNA platform has emerged as the front runner in delivering the first vaccines for emergency use to combat the COVID-19 pandemic,” said Mahima Datla, Biological E.’s managing director.
Messenger ribonucleic acid (mRNA) vaccines prompt the body to make a protein that is part of the virus, triggering an immune response. US companies Pfizer and Moderna use mRNA technology in their COVID-19 shots.
The drug regulator has approved clinical trials of another mRNA vaccine developed by local firm Gennova Biopharmaceuticals, and the government has said it will fund the studies.
Providence Therapeutics Announces Very Favorable Interim Phase 1 Trial Data for PTX-COVID19-B, its mRNA Vaccine Against COVID-19
CALGARY, AB, May 12, 2021 / – Providence Therapeutics Holdings Inc. (“Providence”) announced today very favorable interim clinical data of PTX-COVID19-B, its vaccine candidate against SARS-CoV-2 (“COVID-19”), from its Phase 1 study entitled “PRO-CL-001, A Phase 1, First-in-Human, Observer-Blinded, Randomized, Placebo Controlled, Ascending Dose Study to Evaluate the Safety, Tolerability, and Immunogenicity of PTX-COVID19-B Vaccine in Healthy Seronegative Adults Aged 18-64” (the “Phase 1 Study”), which found that PTX-COVID19-B met Providence’s target results for safety, tolerability, and immunogenicity in the participants of the Phase 1 Study.
Highlights from Providence Therapeutics’ “Phase 1 Study”:
- PTX-COVID19-B was generally safe and well tolerated
- PTX-COVID19-B exhibited strong virus neutralization capability across the 16µg, 40µg and 100µg dose cohorts
- PTX-COVID19-B 40µg dose was selected for Phase 2 study
- PTX-COVID19-B will be evaluated in additional Phase 1 population cohorts
The Phase 1 Study was designed with dose-escalations and was performed in seronegative adult subjects without evidence of recent exposure to COVID-19. The subjects were randomized to receive either the PTX-COVID19-B vaccine or a placebo in a 3:1 ratio. A total of 60 subjects participated in the Phase 1 Study.
The overall results of the Phase 1 Study are that PTX-COVID19-B was safe and well tolerated at the three dose levels of 16µg, 40µg and 100µg. Adverse events identified in the Phase 1 Study were generally mild to moderate in severity, self-resolving and transient. There were no serious adverse events reported in the Phase 1 Study. The most common adverse event reported in the Phase 1 Study was redness and pain at the injection site. Systemic reactions reported in the Phase 1 Study were generally mild to moderate and well tolerated with headache being the most common reaction reported. The reported adverse events of the Phase 1 Study were in line with the expectations of management of Providence as they compare very favorably to the adverse events data published on other mRNA vaccines for COVID-19 that have been approved for use by various health authorities around the world.
Based on the results of the Phase 1 Study, Providence intends to use a 40µg dose for the Phase 2 study of PTX-COVID19-B that is anticipated to be initiated in June 2021. Additional Phase 1 studies in adolescent and elderly populations are also planned to be undertaken by Providence.
PTX-COVID19-B vaccination induced high anti-S IgG antibodies:
Participants in the Phase 1 Study were vaccinated on day zero and day twenty-eight. Plasma samples were collected on day 1, day 8, day 28 (prior to the participant receiving the second dose), and day 42 to determine levels of IgG anti-S protein using electrochemiluminescence (“ECL”) assays from Meso Scale Discovery (“MSD”). Study participants in all three vaccine dose cohorts of the Phase 1 Study developed a strong IgG antibody response against Spike protein that was detected by day 28 and enhanced by day 42. No antibodies against S protein were detected in participants in the Phase 1 Study injected with placebo. The highest levels of antibodies were found in the 40 and 100 µg doses. By day 42, PTX-COVID19-B vaccinated participants had more than one log higher antibody levels than convalescent subjects-plasma (indicated in the dotted line) which was evaluated in the same assay.
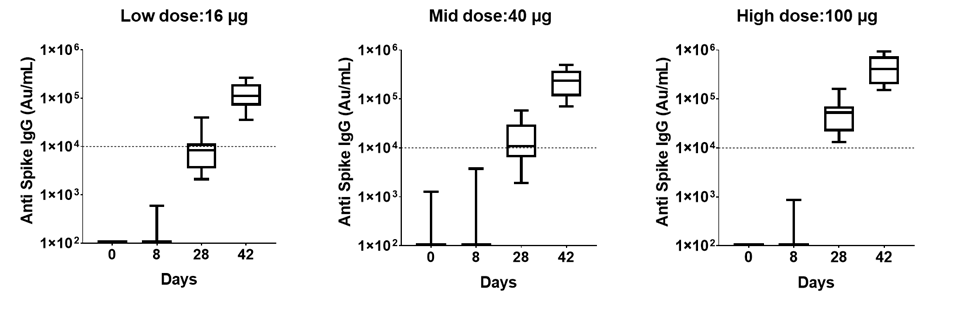
Based on the interim data of the Phase 1 Study, the level of antibodies produced in participants by PTX-COVID19-B compare favorably to the levels of antibodies produced by other mRNA vaccines that have been approved for use against COVID-19 based on the recently published report from Stanford University, where IgG responses in individuals vaccinated with the COVID-19 mRNA vaccine compared to COVID-19 infected patients were evaluated[1].
PTX-COVID19-B vaccination induced high neutralizing antibody levels:
Neutralizing activity from the Phase 1 Study participants’ plasma was evaluated by S-ACE2 MSD assay. The results indicate that the antibodies block the interaction between S protein with the ACE2 receptor and the decrease in ECL signal is used to calculate percentage inhibition of the plasma at the same dilution. All participants in the Phase 1 Study from the 16, 40 and 100 µg dose levels showed blocking activity by day 28 and all of them reached 100% blocking activity by day 42 with samples diluted 1:100 or greater. Moreover, the quantification of the antibody levels in ng/mL with a reference standard showed that all participants in the Phase 1 Study produced neutralizing antibodies by day 28 with the first immunization and increase ten-fold by day 42, two weeks after the administration of the second dose. These results indicate that PTX-COVID19-B induced a strong neutralizing antibody response which compares very favorably to the published results of other mRNA vaccines. Further studies are being conducted by Providence to determine neutralization activity using a pseudo-virus assay.
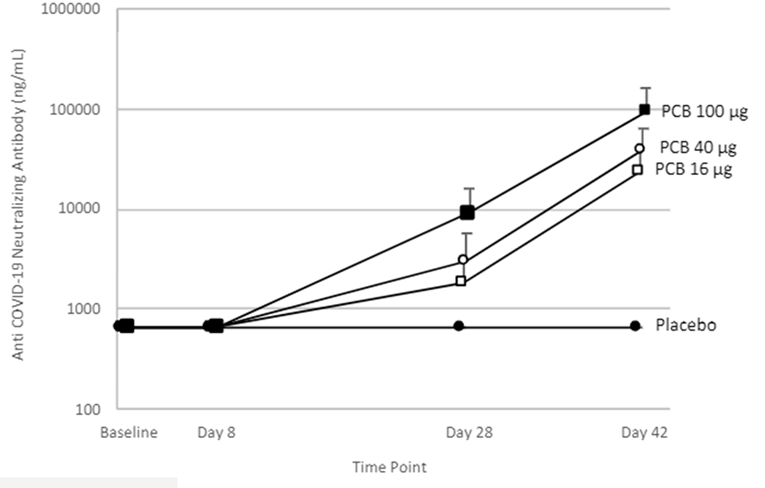
Providence intends to advance a Phase 2 clinical trial in early June 2021, with multiple trial sites in Canada. The Phase 2 clinical trial is anticipated to be structured as a comparator trial using Pfizer/BioNTech vaccine as the positive control.
About Providence Therapeutics
Providence is a leading Canadian clinical stage biotechnology company pioneering mRNA therapeutics and vaccines with operations in Calgary, Alberta and Toronto, Ontario. In response to a worldwide need for a COVID-19 vaccine, Providence expanded its focus beyond oncology therapies and devoted its energy and resources to develop a world-class mRNA vaccine for COVID-19. Providence is focused on serving the needs of Canada, and other countries that may be underserved by large pharmaceutical programs. For more information, please visit providencetherapeutics.com.
References
- ^ “Canadian company urges human trials after COVID-19 vaccine results in mice”. Lethbridge News Now. 5 August 2020. Retrieved 19 March 2021.
- ^ Jump up to:a b c d e f g h Tasker, John Paul (30 April 2021). “COVID-19 vaccine maker Providence says it’s leaving Canada after calls for more federal support go unanswered”. CBC News. Retrieved 1 May 2021.
- ^ Jump up to:a b c Stephenson, Amanda (26 January 2021). “Made-in-Canada COVID vaccine to be manufactured in Calgary”. Calgary Herald. Retrieved 22 March 2021.
- ^ Clinical trial number NCT04765436 for “PTX-COVID19-B, an mRNA Humoral Vaccine, is Intended for Prevention of COVID-19 in a General Population. This Study is Designed to Evaluate Safety, Tolerability, and Immunogenicity of PTX-COVID19-B Vaccine in Healthy Seronegative Adults Aged 18-64” at ClinicalTrials.gov
- ^ “Providence Therapeutics Holdings Inc: PTX-COVID19-B”. Montreal: McGill University. Retrieved 19 March 2021.
- ^ “Made-in-Canada coronavirus vaccine starts human clinical trials”. Canadian Broadcasting Corporation. 26 January 2021.
- ^ Jump up to:a b “Company Profile”. PitchBook.
- ^ Jump up to:a b “Company Profile”. DNB.
- ^ Jump up to:a b Code, Jillian (5 February 2021). “‘Do something’ Made-In-Canada vaccine CEO pleads for federal government to respond”. CTV News. Calgary, Alberta. Retrieved 22 March 2021.
- ^ Jump up to:a b Fieldberg, Alesia (26 January 2021). “Providence Therapeutics begins first clinical trials of Canadian-made COVID-19 vaccine”. CTV. Retrieved 2 May 2021.
- ^ Jump up to:a b c d “Manitoba Supports Made-In-Canada COVID-19 Vaccine to Protect Manitobans” (Press release). 11 February 2021. Retrieved 3 May 2021.
- ^ Providence Therapeutics Holdings Inc.: a Phase I, First-in-Human, Observer-Blinded, Randomized, Placebo Controlled, Ascending Dose Study to Evaluate the Safety, Tolerability, and Immunogenicity of PTX-COVID19-B Vaccine in Healthy Seronegative Adults Aged 18-64 (Report). Clinical Trials via U.S. National Library of Medicine. 19 February 2021. Retrieved 1 May2021.
- ^ Jump up to:a b c Gibson, Shane (11 February 2021). “Manitoba agrees to purchase 2M doses of Providence Therapeutics coronavirus vaccine”. Global News. Retrieved 2 May 2021.
- ^ “Providence Therapeutics begins first clinical trials of Canadian-made COVID-19 vaccine”. CTV. Retrieved 2 May 2021.
- ^ Jump up to:a b c Evidence (PDF), 43rd Parliament, 2nd Session. Standing Committee on International Trade, 29 April 2021, retrieved 2 May2021
- ^ Sorenson, Brad (5 February 2021). “An Open Letter to the Government of Canada”. Retrieved 3 May 2021.
- ^ Dyer, Steven. “‘Canada had an opportunity’, Calgary company explores taking vaccine development out of Canada”. CTV. Retrieved 2 May 2021.
Vaccine description | |
---|---|
Target | SARS-CoV-2 |
Vaccine type | mRNA |
Clinical data | |
Routes of administration | Intramuscular |
Part of a series on the |
COVID-19 pandemic |
---|
COVID-19 (disease)SARS-CoV-2 (virus) |
showTimeline |
showLocations |
showInternational response |
showMedical response |
showImpact |
COVID-19 portal |
////////PTX-COVID19-B, canada, hyderabad, providence, Gennova Biopharmaceuticals, biological e, COVID-19, SARS-CoV-2 , corona virus, covid 19, phase 1

NEW DRUG APPROVALS
ONE TIME
$10.00
NOVAWAX, NVX-CoV2373,

NOVAWAX
SARS-CoV-2 rS Nanoparticle Vaccine
MCDC OTA agreement number W15QKN-16-9-1002
Novavax COVID-19 vaccine, Coronavirus disease 19 infection
SARS-CoV-2 rS, TAK 019
Novavax, Inc. is an American vaccine development company headquartered in Gaithersburg, Maryland, with additional facilities in Rockville, Maryland and Uppsala, Sweden. As of 2020, it had an ongoing Phase III clinical trial in older adults for its candidate vaccine for seasonal influenza, NanoFlu and a candidate vaccine (NVX-CoV2373) for prevention of COVID-19.
NVX-CoV2373 is a SARS-CoV-2 rS vaccine candidate and was shown to have high immunogenicity in studies. The vaccine is created from the genetic sequence of COVID-19 and the antigen derived from the virus spike protein is generated using recombinant nanoparticle technology. The vaccine was developed and tested by Novavax. As of May 2020, the company is pursuing a Phase 1 clinical trial (NCT04368988) to test the vaccine.
History
Novavax was founded in 1987. It focused principally on experimental vaccine development, but did not achieve a successful launch up to 2021.[4]
In June 2013, Novavax acquired the Matrix-M adjuvant platform with the purchase of Swedish company Isconova AB and renamed its new subsidiary Novavax AB.[5]
In 2015, the company received an $89 million grant from the Bill & Melinda Gates Foundation to support the development of a vaccine against human respiratory syncytial virus for infants via maternal immunization.[6][7][8][9]
In March 2015 the company completed a Phase I trial for its Ebola vaccine candidate,[10] as well as a phase II study in adults for its RSV vaccine, which would become ResVax.[11] The ResVax trial was encouraging as it showed significant efficacy against RSV infection.[11]
2016 saw the company’s first phase III trial, the 12,000 adult Resolve trial,[11] for its respiratory syncytial virus vaccine, which would come to be known as ResVax, fail in September.[3] This triggered an eighty-five percent dive in the company’s stock price.[3] Phase II adult trial results also released in 2016 showed a stimulation of antigencity, but failure in efficacy.[11] Evaluation of these results suggested that an alternative dosing strategy might lead to success, leading to plans to run new phase II trials.[3] The company’s difficulties in 2016 led to a three part strategy for 2017: cost reduction through restructuring and the termination of 30% of their workforce; pouring more effort into getting ResVax to market; and beginning clinical trials on a Zika virus vaccine.[3]
Alongside the adult studies of ResVax, the vaccine was also in 2016 being tested against infant RSV infection through the route of maternal immunization.[11]
In 2019, late-stage clinical testing of ResVax, failed for a second time, which resulted in a major downturn in investor confidence and a seventy percent reduction in capital value for the firm.[12][13] As a secondary result, the company was forced to conduct a reverse stock split in order to maintain Nasdaq minimum qualification, meaning it was in risk of being delisted.[13]
The company positions NanoFlu for the unmet need for a more effective vaccine against influenza, particularly in the elderly who often experience serious and sometimes life-threatening complications. In January 2020, it was granted fast track status by the U.S. Food and Drug Administration (FDA) for NanoFlu.
External sponsorships
In 2018, Novavax received a US$89 million research grant from the Bill and Melinda Gates Foundation for development of vaccines for maternal immunization.[14]
In May 2020, Novavax received US$384 million from the Coalition for Epidemic Preparedness Innovations to fund early-stage evaluation in healthy adults of the company’s COVID-19 vaccine candidate NVX-CoV2373 and to develop resources in preparation for large-scale manufacturing, if the vaccine proves successful.[15] CEPI had already invested $4 million in March.[15]
Drugs in development
ResVax is a nanoparticle-based treatment using a recombinant F lipoprotein or saponin, “extracted from the Quillaja saponaria [or?] Molina bark together with cholesterol and phospholipid.”[16] It is aimed at stimulating resistance to respiratory syncytial virus infection, targeting both adult and infant populations.[11]
In January 2020, Novavax was given Fast Track status by the FDA to expedite the review process for NanoFlu, a candidate influenze vaccine undergoing a Phase III clinical trial scheduled for completion by mid-2020.[17]
COVID-19 vaccine candidate
See also: NVX-CoV2373 and COVID-19 vaccine
In January 2020, Novavax announced development of a vaccine candidate, named NVX-CoV2373, to establish immunity to SARS-CoV-2.[18] NVX-CoV2373 is a protein subunit vaccine that contains the spike protein of the SARS-CoV-2 virus.[19] Novavax’s work is in competition for vaccine development among dozens of other companies.
In January 2021, the company released phase 3 trials showing that it has 89% efficacy against Covid-19, and also provides strong immunity against new variants.[20] It has applied for emergency use in the US and UK but will be distributed in the UK first.Novavax COVID-19 Vaccine Demonstrates 89.3% Efficacy in UK Phase 3 TrialJan 28, 2021 at 4:05 PM ESTDownload PDF
First to Demonstrate Clinical Efficacy Against COVID-19 and Both UK and South Africa Variants
- Strong efficacy in Phase 3 UK trial with over 50% of cases attributable to the now-predominant UK variant and the remainder attributable to COVID-19 virus
- Clinical efficacy demonstrated in Phase 2b South Africa trial with over 90% of sequenced cases attributable to prevalent South Africa escape variant
- Company to host investor conference call today at 4:30pm ET
GAITHERSBURG, Md., Jan. 28, 2021 (GLOBE NEWSWIRE) — Novavax, Inc. (Nasdaq: NVAX), a biotechnology company developing next-generation vaccines for serious infectious diseases, today announced that NVX-CoV2373, its protein-based COVID-19 vaccine candidate, met the primary endpoint, with a vaccine efficacy of 89.3%, in its Phase 3 clinical trial conducted in the United Kingdom (UK). The study assessed efficacy during a period with high transmission and with a new UK variant strain of the virus emerging and circulating widely. It was conducted in partnership with the UK Government’s Vaccines Taskforce. Novavax also announced successful results of its Phase 2b study conducted in South Africa.
“With today’s results from our UK Phase 3 and South Africa Phase 2b clinical trials, we have now reported data on our COVID-19 vaccine from Phase 1, 2 and 3 trials involving over 20,000 participants. In addition, our PREVENT-19 US and Mexico clinical trial has randomized over 16,000 participants toward our enrollment goal of 30,000. NVX-CoV2373 is the first vaccine to demonstrate not only high clinical efficacy against COVID-19 but also significant clinical efficacy against both the rapidly emerging UK and South Africa variants,” said Stanley C. Erck, President and Chief Executive Officer, Novavax. “NVX-CoV2373 has the potential to play an important role in solving this global public health crisis. We look forward to continuing to work with our partners, collaborators, investigators and regulators around the world to make the vaccine available as quickly as possible.”
NVX-CoV2373 contains a full-length, prefusion spike protein made using Novavax’ recombinant nanoparticle technology and the company’s proprietary saponin-based Matrix-M™ adjuvant. The purified protein is encoded by the genetic sequence of the SARS-CoV-2 spike (S) protein and is produced in insect cells. It can neither cause COVID-19 nor can it replicate, is stable at 2°C to 8°C (refrigerated) and is shipped in a ready-to-use liquid formulation that permits distribution using existing vaccine supply chain channels.
UK Phase 3 Results: 89.3% Efficacy
The study enrolled more than 15,000 participants between 18-84 years of age, including 27% over the age of 65. The primary endpoint of the UK Phase 3 clinical trial is based on the first occurrence of PCR-confirmed symptomatic (mild, moderate or severe) COVID-19 with onset at least 7 days after the second study vaccination in serologically negative (to SARS-CoV-2) adult participants at baseline.
The first interim analysis is based on 62 cases, of which 56 cases of COVID-19 were observed in the placebo group versus 6 cases observed in the NVX-CoV2373 group, resulting in a point estimate of vaccine efficacy of 89.3% (95% CI: 75.2 – 95.4). Of the 62 cases, 61 were mild or moderate, and 1 was severe (in placebo group).
Preliminary analysis indicates that the UK variant strain that was increasingly prevalent was detected in over 50% of the PCR-confirmed symptomatic cases (32 UK variant, 24 non-variant, 6 unknown). Based on PCR performed on strains from 56 of the 62 cases, efficacy by strain was calculated to be 95.6% against the original COVID-19 strain and 85.6% against the UK variant strain [post hoc].
The interim analysis included a preliminary review of the safety database, which showed that severe, serious, and medically attended adverse events occurred at low levels and were balanced between vaccine and placebo groups.
“These are spectacular results, and we are very pleased to have helped Novavax with the development of this vaccine. The efficacy shown against the emerging variants is also extremely encouraging. This is an incredible achievement that will ensure we can protect individuals in the UK and the rest of the world from this virus,” said Clive Dix, Chair, UK Vaccine Taskforce.
Novavax expects to share further details of the UK trial results as additional data become available. Additional analysis on both trials is ongoing and will be shared via prepublication servers as well as submitted to a peer-reviewed journal for publication. The company initiated a rolling submission to the United Kingdom’s regulatory agency, the MHRA, in mid-January.
South Africa Results: Approximately 90% of COVID-19 cases attributed to South Africa escape variant
In the South Africa Phase 2b clinical trial, 60% efficacy (95% CI: 19.9 – 80.1) for the prevention of mild, moderate and severe COVID-19 disease was observed in the 94% of the study population that was HIV-negative. Twenty-nine cases were observed in the placebo group and 15 in the vaccine group. One severe case occurred in the placebo group and all other cases were mild or moderate. The clinical trial also achieved its primary efficacy endpoint in the overall trial population, including HIV-positive and HIV-negative subjects (efficacy of 49.4%; 95% CI: 6.1 – 72.8).
This study enrolled over 4,400 patients beginning in August 2020, with COVID-19 cases counted from September through mid-January. During this time, the triple mutant variant, which contains three critical mutations in the receptor binding domain (RBD) and multiple mutations outside the RBD, was widely circulating in South Africa. Preliminary sequencing data is available for 27 of 44 COVID-19 events; of these, 92.6% (25 out of 27 cases) were the South Africa escape variant.
Importantly in this trial, approximately 1/3 of the patients enrolled (but not included in the primary analyses described above) were seropositive, demonstrating prior COVID-19 infection at baseline. Based on temporal epidemiology data in the region, the pre-trial infections are thought to have been caused by the original COVID-19 strain (i.e., non-variant), while the subsequent infections during the study were largely variant virus. These data suggest that prior infection with COVID-19 may not completely protect against subsequent infection by the South Africa escape variant, however, vaccination with NVX-CoV2373 provided significant protection.
“The 60% reduced risk against COVID-19 illness in vaccinated individuals in South Africans underscores the value of this vaccine to prevent illness from the highly worrisome variant currently circulating in South Africa, and which is spreading globally. This is the first COVID-19 vaccine for which we now have objective evidence that it protects against the variant dominating in South Africa,” says Professor Shabir Maddi, Executive Director of the Vaccines and Infectious Diseases Analytics Research Unit (VIDA) at Wits, and principal investigator in the Novavax COVID-19 vaccine trial in South Africa. “I am encouraged to see that Novavax plans to immediately begin clinical development on a vaccine specifically targeted to the variant, which together with the current vaccine is likely to form the cornerstone of the fight against COVID-19.”
Novavax initiated development of new constructs against the emerging strains in early January and expects to select ideal candidates for a booster and/or combination bivalent vaccine for the new strains in the coming days. The company plans to initiate clinical testing of these new vaccines in the second quarter of this year.
“A primary benefit of our adjuvanted platform is that it uses a very small amount of antigen, enabling the rapid creation and large-scale production of combination vaccine candidates that could potentially address multiple circulating strains of COVID-19,” said Gregory M. Glenn, M.D., President of Research and Development, Novavax. “Combined with the safety profile that has been observed in our studies to-date with our COVID-19 vaccine, as well as prior studies in influenza, we are optimistic about our ability to rapidly adapt to evolving conditions.”
The Coalition for Epidemic Preparedness Innovations (CEPI) funded the manufacturing of doses of NVX-CoV2373 for this Phase 2b clinical trial, which was supported in part by a $15 million grant from the Bill & Melinda Gates Foundation.
Significant progress on PREVENT-19 Clinical Trial in US and Mexico
To date, PREVENT-19 has randomized over 16,000 participants and expects to complete our targeted enrollment of 30,000 patients in the first half of February. PREVENT-19 is being conducted with support from the U.S. government partnership formerly known as Operation Warp Speed, which includes the Department of Defense, the Biomedical Advanced Research and Development Authority (BARDA), part of the U.S. Department of Health and Human Services (HHS) Office of the Assistant Secretary for Preparedness and Response, and the National Institute of Allergy and Infectious Diseases (NIAID), part of the National Institutes of Health (NIH) at HHS. BARDA is also providing up to $1.75 billion under a Department of Defense agreement.
PREVENT-19 (the PRE-fusion protein subunit Vaccine Efficacy Novavax Trial | COVID-19) is a Phase 3, randomized, placebo-controlled, observer-blinded study in the US and Mexico to evaluate the efficacy, safety and immunogenicity of NVX-CoV2373 with Matrix-M in up to 30,000 subjects 18 years of age and older compared with placebo. The trial design has been harmonized to align with other Phase 3 trials conducted under the auspices of Operation Warp Speed, including the use of a single external independent Data and Safety Monitoring Board to evaluate safety and conduct an unblinded review when predetermined interim analysis events are reached.
The trial’s primary endpoint is the prevention of PCR-confirmed, symptomatic COVID-19. The key secondary endpoint is the prevention of PCR-confirmed, symptomatic moderate or severe COVID-19. Both endpoints will be assessed at least seven days after the second study vaccination in volunteers who have not been previously infected with SARS-CoV-2.
Conference Call
Novavax will host a conference call today at 4:30pm ET. The dial-in numbers for the conference call are (877) 212-6076 (Domestic) or (707) 287-9331 (International), passcode 7470222. A replay of the conference call will be available starting at 7:30 p.m. ET on January 28, 2021 until 7:30 p.m. ET on February 4, 2021. To access the replay by telephone, dial (855) 859-2056 (Domestic) or (404) 537-3406 (International) and use passcode 7470222.
A webcast of the conference call can also be accessed on the Novavax website at novavax.com/events. A replay of the webcast will be available on the Novavax website until April 28, 2021.
About NVX-CoV2373
NVX-CoV2373 is a protein-based vaccine candidate engineered from the genetic sequence of SARS-CoV-2, the virus that causes COVID-19 disease. NVX-CoV2373 was created using Novavax’ recombinant nanoparticle technology to generate antigen derived from the coronavirus spike (S) protein and is adjuvanted with Novavax’ patented saponin-based Matrix-M™ to enhance the immune response and stimulate high levels of neutralizing antibodies. NVX-CoV2373 contains purified protein antigen and can neither replicate, nor can it cause COVID-19. Over 37,000 participants have participated to date across four different clinical studies in five countries. NVX-CoV2373 is currently being evaluated in two pivotal Phase 3 trials: a trial in the U.K that completed enrollment in November and the PREVENT-19 trial in the U.S. and Mexico that began in December.
About Matrix-M™
Novavax’ patented saponin-based Matrix-M™ adjuvant has demonstrated a potent and well-tolerated effect by stimulating the entry of antigen presenting cells into the injection site and enhancing antigen presentation in local lymph nodes, boosting immune response.
About Novavax
Novavax, Inc. (Nasdaq: NVAX) is a biotechnology company that promotes improved health globally through the discovery, development and commercialization of innovative vaccines to prevent serious infectious diseases. The company’s proprietary recombinant technology platform combines the power and speed of genetic engineering to efficiently produce highly immunogenic nanoparticles designed to address urgent global health needs. Novavax is conducting late-stage clinical trials for NVX-CoV2373, its vaccine candidate against SARS-CoV-2, the virus that causes COVID-19. NanoFlu™, its quadrivalent influenza nanoparticle vaccine, met all primary objectives in its pivotal Phase 3 clinical trial in older adults and will be advanced for regulatory submission. Both vaccine candidates incorporate Novavax’ proprietary saponin-based Matrix-M™ adjuvant to enhance the immune response and stimulate high levels of neutralizing antibodies.
For more information, visit www.novavax.com and connect with us on Twitter and LinkedIn.
Candidate: NVX-CoV2373
Category: VAX
Type: Stable, prefusion protein made using Novavax’ proprietary nanoparticle technology, and incorporating its proprietary saponin-based Matrix-M™ adjuvant.
2021 Status: Novavax on March 11 announced final efficacy of 96.4% against mild, moderate and severe disease caused by the original COVID-19 strain in a pivotal Phase III trial in the U.K. of NVX–CoV2373. The study enrolled more than 15,000 participants between 18-84 years of age, including 27% over the age of 65.
The company also announced the complete analysis of its Phase IIb trial in South Africa, showing the vaccine had an efficacy of 55.4% among a cohort of HIV-negative trial participants, and an overall efficacy of 48.6% against predominantly variant strains of SARS-CoV-2 among 147 PCR-positive cases (51 cases in the vaccine group and 96 in the placebo group). Across both trials, NVX-CoV2373 demonstrated 100% protection against severe disease, including all hospitalization and death.
Philippines officials said March 10 that they secured 30 million doses of NVX-CoV2373 through an agreement with the Serum Institute of India, the second vaccine deal signed by the national government, according to Agence France-Presse. The first was with AstraZeneca for 2.6 million doses of its vaccine, developed with Oxford University.
The Novavax vaccine will be available from the third quarter, at a price that has yet to be finalized. The government hopes to secure 148 million doses this year from seven companies—enough for around 70% of its population.
In announcing fourth quarter and full-year 2020 results on March 1, Novavax said it could file for an emergency use authorization with the FDA in the second quarter of 2021. Novavax hopes it can use data from its Phase III U.K. clinical trial in its FDA submission, and expects the FDA to examine data in May, a month after they are reviewed by regulators in the U.K., President and CEO Stanley C. Erck said on CNBC. Should the FDA insist on waiting for U.S. data, the agency may push the review timeline by one or two months, he added.
The company also said that NVX-CoV2373 showed 95.6% efficacy against the original strain of COVID-19 and 85.6% against the UK variant strain, and re-stated an earlier finding that its vaccine met the Phase III trial’s primary endpoint met with an efficacy rate of 89.3%.
Novavax said February 26 that it signed an exclusive license agreement with Takeda Pharmaceutical for Takeda to develop, manufacture, and commercialize NVX-CoV2373 in Japan.
Novavax agreed to transfer the technology for manufacturing of the vaccine antigen and will supply its Matrix-M™ adjuvant to Takeda. Takeda anticipated the capacity to manufacture over 250 million doses of the COVID-19 vaccine per year. Takeda agreed in return to pay Novavax undisclosed payments tied to achieving development and commercial milestones, plus a portion of proceeds from the vaccine.
Takeda also disclosed that it dosed the first participants in a Phase II clinical trial to test the immunogenicity and safety of Novavax’ vaccine candidate in Japanese participants.
Novavax on February 18 announced a memorandum of understanding with Gavi, the Vaccine Alliance (Gavi), to provide 1.1 billion cumulative doses of NVX-CoV2373 for the COVAX Facility. Gavi leads the design and implementation of the COVAX Facility, created to supply vaccines globally, and has committed to working with Novavax to finalize an advance purchase agreement for vaccine supply and global distribution allocation via the COVAX Facility and its partners.
The doses will be manufactured and distributed globally by Novavax and Serum Institute of India (SII), the latter under an existing agreement between Gavi and SII.
Novavax and SK Bioscience said February 15 that they expanded their collaboration and license agreement, with SK finalizing an agreement to supply 40 million doses of NVX-CoV2373 to the government of South Korea beginning in 2021, for an undisclosed price. SK also obtained a license to manufacture and commercialize NVX-CoV2373 for sale to South Korea, as a result of which SK said it will add significant production capacity.
The agreement also calls on Novavax to facilitate technology transfer related to the manufacturing of its protein antigen, its Matrix M adjuvant, and support to SK Bioscience as needed to secure regulatory approval.
Rolling review begins—On February 4, Novavax announced it had begun a rolling review process for authorization of NVX-CoV2373 with several regulatory agencies worldwide, including the FDA, the European Medicines Agency, the U.K. Medicines and Healthcare products Regulatory Agency (MHRA), and Health Canada. The reviews will continue while the company completes its pivotal Phase III trials in the U.S. and U.K., and through initial authorization for emergency use granted under country-specific regulations, and through initial authorization for emergency use.
A day earlier, Novavax executed a binding Heads of Terms agreement with the government of Switzerland to supply 6 million doses of NVX-CoV2373, to the country. Novavax and Switzerland plan to negotiate a final agreement, with initial delivery of vaccine doses slated to ship following successful clinical development and regulatory review.
On January 28, Novavax electrified investors by announcing that its COVID-19 vaccine NVX-CoV2373 showed efficacy of 89.3% in the company’s first analysis of data from a Phase III trial in the U.K., where a variant strain (B.1.1.7) accounted for about half of all positive cases.
However, NVX-CoV2373 achieved only 60% efficacy in a Phase IIb trial in South Africa, where that country’s escape variant of the virus (B.1.351, also known as 20H/501Y.V2) was seen in 90% of cases, Novavax said.
Novavax said January 7 it executed an Advance Purchase Agreement with the Commonwealth of Australia for 51 million doses of NVX-CoV2373 for an undisclosed price, with an option to purchase an additional 10 million doses—finalizing an agreement in principle announced in November 2020. Novavax said it will work with Australia’s Therapeutics Goods Administration (TGA), to obtain approvals upon showing efficacy in clinical studies. The company aims to deliver initial doses by mid-2021.
2020 Status: Phase III trial launched—Novavax said December 28 that it launched the pivotal Phase III PREVENT-19 trial (NCT04611802) in the U.S. and Mexico to evaluate the efficacy, safety and immunogenicity of NVX-CoV2373. The randomized, placebo-controlled, observer-blinded study will assess the efficacy, safety and immunogenicity of NVX-CoV2373 in up to 30,000 participants 18 years of age and older compared with placebo. The trial’s primary endpoint is the prevention of PCR-confirmed, symptomatic COVID-19. The key secondary endpoint is the prevention of PCR-confirmed, symptomatic moderate or severe COVID-19. Both endpoints will be assessed at least seven days after the second study vaccination in volunteers who have not been previously infected with SARS-CoV-2.
Two thirds of the participants will be assigned to randomly receive two intramuscular injections of the vaccine, administered 21 days apart, while one third of the trial participants will receive placebo. Trial sites were selected in locations where transmission rates are currently high, to accelerate the accumulation of positive cases that could show efficacy. Participants will be followed for 24 months following the second injection
PREVENT-19 is being conducted with support from federal agencies involved in Operation Warp Speed, the Trump administration’s effort to promote development and distribution of COVID-19 vaccines and drugs. Those agencies include the Department of Defense (DoD), the NIH’s National Institute of Allergy and Infectious Diseases (NIAID), and the Biomedical Advanced Research and Development Authority (BARDA)—which has committed up to $1.6 billion to Novavax under a DoD agreement (identifier MCDC OTA agreement number W15QKN-16-9-1002).
Novavax is also conducting a pivotal Phase III study in the United Kingdom, a Phase IIb safety and efficacy study in South Africa, and an ongoing Phase I/II trial in the U.S. and Australia. Data from these trials are expected as soon as early first quarter 2021, though timing will depend on transmission rates in the regions, the company said.
Novavax said November 9 that the FDA granted its Fast Track designation for NVX-CoV2373. By the end of November, the company expected to finish enrollment in its Phase III U.K. trial, with interim data in that study expected as soon as early first quarter 2021.
Five days earlier, Novavax signed a non-binding Heads of Terms document with the Australian government to supply 40 million doses of NVX-CoV2373 to Australia starting as early as the first half of 2021, subject to the successful completion of Phase III clinical development and approval of the vaccine by Australia’s Therapeutic Goods Administration (TGA). The vaccine regimen is expected to require two doses per individual, administered 21 days apart.
Australia joins the U.S., the U.K., and Canada in signing direct supply agreements with Novavax. The company is supplying doses in Japan, South Korea, and India through partnerships. Australian clinical researchers led the global Phase I clinical trial in August, which involved 131 Australians across two trial sites (Melbourne and Brisbane). Also, approximately 690 Australians have participated in the Phase II arm of the clinical trial, which has been conducted across up to 40 sites in Australia and the U.S.
Novavax joined officials in its headquarters city of Gaithersburg, MD, on November 2 to announce expansion plans. The company plans to take 122,000 square feet of space at 700 Quince Orchard Road, and has committed to adding at least 400 local jobs, nearly doubling its current workforce of 450 worldwide. Most of the new jobs are expected to be added b March 2021.
Maryland’s Department of Commerce—which has prioritized assistance to life sciences companies—approved a $2 million conditional loan tied to job creation and capital investment. The state has also approved a $200,000 Partnership for Workforce Quality training grant, and the company is eligible for several tax credits, including the Job Creation Tax Credit and More Jobs for Marylanders.
Additionally, Montgomery County has approved a $500,000 grant tied to job creation and capital investment, while the City of Gaithersburg said it will approve a grant of up to $50,000 from its Economic Development Opportunity Fund. The city accelerated its planning approval process to accommodate Novavax’ timeline, given the company’s role in fighting COVID-19 and resulting assistance from Operation Warp Speed, the Trump administration’s effort to accelerate development of COVID-19 vaccines.
On October 27, Novavax said that it had enrolled 5,500 volunteers in the Phase III U.K. trial, which has been expanded from 10,000 to 15,000 volunteers. The increased enrollment “is likely to facilitate assessment of safety and efficacy in a shorter time period,” according to the company.
The trial, which is being conducted with the U.K. Government’s Vaccines Taskforce, was launched in September and is expected to be fully enrolled by the end of November, with interim data expected by early first quarter 2021, depending on the overall COVID-19 attack rate. Novavax has posted the protocol for the Phase III U.K. trial online. The protocol calls for unblinding of data once 152 participants have achieved mild, moderate or severe endpoints. Two interim analyses are planned upon occurrence of 66 and 110 endpoints.
Novavax also said it expects to launch a second Phase III trial designed to enroll up to 30,000 participants in the U.S. and Mexico by the end of November—a study funded through the U.S. government’s Operation Warp Speed program. The patient population will reflect proportional representation of diverse populations most vulnerable to COVID-19, across race/ethnicity, age, and co-morbidities.
The company cited progress toward large-scale manufacturing while acknowledging delays from original timeframe estimates. Novavax said it will use its contract manufacturing site at FUJIFILM Diosynth Biotechnologies’ Morrisville, NC facility to produce material for the U.S. trial.
On September 25, Novavax entered into a non-exclusive agreement with Endo International subsidiary Par Sterile Products to provide fill-finish manufacturing services at its plant in Rochester, MI, for NVX-CoV2373. Under the agreement, whose value was not disclosed, the Rochester facility has begun production of NVX-CoV2373 final drug product, with initial batches to be used in Novavax’ Phase III clinical trial in the U.S. Par Sterile will also fill-finish NVX-CoV2373 vaccine intended for commercial distribution in the U.S.
A day earlier, Novavax launched the U.K. trial. The randomized, placebo-controlled, observer-blinded study to evaluate the efficacy, safety and immunogenicity of NVX-CoV2373 with Matrix-M in up to 10,000 subjects 18-84 years of age, with and without “relevant” comorbidities, over the following four to six weeks, Novavax said. Half the participants will receive two intramuscular injections of vaccine comprising 5 µg of protein antigen with 50 µg Matrix‑M adjuvant, 21 days apart, while half of the trial participants will receive placebo. At least 25% of the study population will be over age 65.
The trial’s first primary endpoint is first occurrence of PCR-confirmed symptomatic COVID-19 with onset at least seven days after the second study vaccination in volunteers who have not been previously infected with SARS-CoV-2. The second primary endpoint is first occurrence of PCR-confirmed symptomatic moderate or severe COVID-19 with onset at least seven days after the second study vaccination in volunteers who have not been previously infected with SARS-CoV-2
“The data from this trial is expected to support regulatory submissions for licensure in the UK, EU and other countries,” stated Gregory M. Glenn, M.D., President, Research and Development at Novavax.
Maryland Gov. Larry Hogan joined state Secretary of Commerce Kelly M. Schulz and local officials in marking the launch of Phase III studies with a tour of the company’s facilities in Gaithersburg: “The coronavirus vaccine candidate that’s been developed by Novavax is one of the most promising in the country, if not the world.”
On August 31, Novavax reached an agreement in principle with the government of Canada to supply up to 76 million doses of NVX-CoV2373. The value was not disclosed. Novavax and Canada did say that they expect to finalize an advance purchase agreement under which Novavax will agree to supply doses of NVX-CoV2373 to Canada beginning as early as the second quarter of 2021.
The purchase arrangement will be subject to licensure of the NVX-CoV2373 by Health Canada, Novavax said. The vaccine is in multiple Phase II clinical trials: On August 24, Novavax said the first volunteers had been enrolled in the Phase II portion of its ongoing Phase I/II clinical trial (NCT04368988), designed to evaluate the immunogenicity and safety of two doses of of NVX-CoV2373 (5 and 25 µg) with and without 50 µg of Matrix‑M™ adjuvant in up to 1,500 volunteers ages 18-84.
The randomized, placebo-controlled, observer-blinded study is designed to assess two dose sizes (5 and 25 µg) of NVX-CoV2373, each with 50 µg of Matrix‑M. Unlike the Phase I portion, the Phase II portion will include older adults 60-84 years of age as approximately half of the trial’s population. Secondary objectives include preliminary evaluation of efficacy. The trial will be conducted at up to 40 sites in the U.S. and Australia, Novovax said.
NVX-CoV2373 is in a pair of Phase II trials launched in August—including a Phase IIb study in South Africa to assess efficacy, and a Phase II safety and immunogenicity study in the U.S. and Australia.
On August 14, the U.K. government agreed to purchase 60 million doses of NVX-CoV2373 from the company, and support its planned Phase III clinical trial in the U.K., through an agreement whose value was not disclosed. The doses are set to be manufactured as early as the first quarter of 2021.
The trial will be designed to evaluate the ability of NVX-CoV2373 to protect against symptomatic COVID-19 disease as well as evaluate antibody and T-cell responses. The randomized, double-blind, placebo-controlled efficacy study will enroll approximately 9,000 adults 18-85 years of age in the U.K., and is expected to start in the third quarter.
Novavax also said it will expand its collaboration with FUJIFILM Diosynth Biotechnologies (FDB), which will manufacture the antigen component of NVX-CoV2373 from its Billingham, Stockton-on-Tees site in the U.K., as well as at U.S. sites in Morrisville, NC, and College Station, TX. FDB’s U.K. sitevis expected to produce up to 180 million doses annually.
On August 13, Novavax said it signed a development and supply agreement for the antigen component of NVX-CoV2373 with Seoul-based SK bioscience, a vaccine business subsidiary of SK Group. The agreement calls for supply to global markets that include the COVAX Facility, co-led by Gavi, the Coalition for Epidemic Preparedness Innovations (CEPI) and the World Health Organization.
Novavax and SK signed a letter of intent with South Korea’s Ministry of Health and Welfare to work toward broad and equitable access to NVX-CoV2373 worldwide, as well as to make the vaccine available in South Korea. SK bioscience agreed to manufacture the vaccine antigen component for use in the final drug product globally during the pandemic, at its vaccine facility in Andong L-house, South Korea, beginning in August. The value of the agreement was not disclosed.
On August 7, Novavax licensed its COVID-19 vaccine technology to Takeda Pharmaceutical through a partnership by which Takeda will develop, manufacture, and commercialize NVX‑CoV2373 in Japan, using Matrix-M adjuvant to be supplied by Novavax. Takeda will also be responsible for regulatory submission to Japan’s Ministry of Health, Labour and Welfare (MHLW).
MHLW agreed to provide funding to Takeda—the amount was not disclosed in the companies’ announcement—for technology transfer, establishment of infrastructure, and scale-up of manufacturing. Takeda said it anticipated the capacity to manufacture over 250 million doses of NVX‑CoV2373 per year.
Five days earlier, Serum Institute of India agreed to license rights from Novavax to NVX‑CoV2373 for development and commercialization in India as well as low- and middle-income countries (LMIC), through an agreement whose value was not disclosed. Novavax retains rights to NVX-CoV2373 elsewhere in the world.
Novavax and Serum Institute of India agreed to partner on clinical development, co-formulation, filling and finishing and commercialization of NVX-CoV2373. Serum Institute will oversee regulatory submissions and marketing authorizations in regions covered by the collaboration. Novavax agreed to provide both vaccine antigen and Matrix‑M adjuvant, while the partners said they were in talks to have the Serum Institute manufacture vaccine antigen in India. Novavax and Seerum Institute plan to split the revenue from the sale of product, net of agreed costs.
A day earlier, Novavax announced positive results from the Phase I portion of its Phase I/II clinical trial (NCT04368988), designed to evaluate two doses of NVX-CoV2373 (5 and 25 µg) with and without Matrix‑M™ adjuvant in 131 healthy adults ages 18-59. NVX-CoV2373, adjuvanted with Matrix-M, elicited robust antibody responses numerically superior to human convalescent sera, according to data submitted for peer-review to a scientific journal.
All participants developed anti-spike IgG antibodies after a single dose of vaccine, Novavax said, many also developing wild-type virus neutralizing antibody responses. After the second dose, all participants developed wild-type virus neutralizing antibody responses. Both anti-spike IgG and viral neutralization responses compared favorably to responses from patients with clinically significant COVID‑19 disease, the company said—adding that IgG antibody response was highly correlated with neutralization titers, showing that a significant proportion of antibodies were functional.
For both dosages of NVX‑CoV2373 with adjuvant, the 5 µg dose performed “comparably” with the 25 µg dose, Novavax said. NVX‑CoV2373 also induced antigen-specific polyfunctional CD4+ T cell responses with a strong bias toward the Th1 phenotype (IFN-g, IL-2, and TNF-a).
Based on an interim analysis of Phase I safety and immunogenicity data, the trial was expanded to Phase II clinical trials in multiple countries, including the U.S. The trial—which began in Australia in May—is being funded by up-to $388 million in funding from the Coalition for Epidemic Preparedness Innovations (CEPI). If the Phase I/II trial is successful, CEPI said, it anticipates supporting further clinical development that would advance NVX-CoV2373 through to licensure.
On July 23, Novavax joined FDB to announce that FDB will manufacture bulk drug substance for NVX-CoV2373, under an agreement whose value was not disclosed. FDB’s site in Morrisville, NC has begun production of the first batch of NVX-CoV2373. Batches produced at FDB’s Morrisville site will be used in Novavax’s planned pivotal Phase III clinical trial, designed to assess NVX-CoV2373 in up to 30,000 participants, and set to start this fall.
The Phase III trial is among R&D efforts to be funded through the $1.6 billion awarded in July to Novavax through President Donald Trump’s “Operation Warp Speed” program toward late-stage clinical trials and large-scale manufacturing to produce 100 million doses of its COVID-19 vaccine by year’s end. Novavax said the funding will enable it to complete late-stage clinical studies aimed at evaluating the safety and efficacy of NVX-CoV2373.
In June, Novavax said biotech investor and executive David Mott was joining its board as an independent director, after recently acquiring nearly 65,000 shares of the company’s common stock. Also, Novavax was awarded a $60 million contract by the U.S. Department of Defense (DoD) for the manufacturing of NVX‑CoV2373. Through the Defense Health Program, the Joint Program Executive Office for Chemical, Biological, Radiological and Nuclear Defense Enabling Biotechnologies (JPEO-CBRND-EB) agreed to support production of several vaccine components to be manufactured in the U.S. Novavax plans to deliver this year for DoD 10 million doses of NVX‑CoV2373 that could be used in Phase II/III trials, or under an Emergency Use Authorization (EUA) if approved by the FDA.
Also in June, AGC Biologics said it will partner with Novavax on large-scale GMP production of Matrix-M– significantly increasing Novavax’ capacity to deliver doses in 2020 and 2021—through an agreement whose value was not disclosed. And Novavax joined The PolyPeptide Group to announce large-scale GMP production by the global CDMO of two unspecified key intermediate components used in the production of Matrix-M.
In May, Novavax acquired Praha Vaccines from the India-based Cyrus Poonawalla Group for $167 million cash, in a deal designed to ramp up Novavax’s manufacturing capacity for NVX-CoV2373. Praha Vaccines’ assets include a 150,000-square foot vaccine and biologics manufacturing facility and other support buildings in Bohumil, Czech Republic. Novavax said the Bohumil facility is expected to deliver an annual capacity of over 1 billion doses of antigen starting in 2021 for the COVID-19 vaccine.
The Bohumil facility is completing renovations that include the addition of Biosafety Level-3 (BSL-3) capabilities. The site’s approximately 150 employees with “significant experience” in vaccine manufacturing and support have joined Novavax, the company said.
On May 11, Novavax joined CEPI in announcing up to $384 million in additional funding for the company toward clinical development and large-scale manufacturing of NVX-CoV2373. CEPI agreed to fund preclinical as well as Phase I and Phase II studies of NVX-CoV2373. The funding multiplied CEPI’s initial $4 million investment in the vaccine candidate, made two months earlier. Novavax’s total $388 million in CEPI funding accounted for 87% of the total $446 million awarded by the Coalition toward COVID-19 vaccine R&D as of that date.
Novavax identified its COVID-19 vaccine candidate in April. The company said NVX-CoV2373 was shown to be highly immunogenic in animal models measuring spike protein-specific antibodies, antibodies that block the binding of the spike protein to the receptor, and wild-type virus neutralizing antibodies. High levels of spike protein-specific antibodies with ACE-2 human receptor binding domain blocking activity and SARS-CoV-2 wild-type virus neutralizing antibodies were also seen after a single immunization.
In March, Emergent Biosolutions disclosed it retained an option to allocate manufacturing capacity for an expanded COVID-19 program under an agreement with Novavax to provide “molecule-to-market” contract development and manufacturing (CDMO) services to produce Novavax’s NanoFlu™, its recombinant quadrivalent seasonal influenza vaccine candidate.
Earlier in March, Emergent announced similar services to support clinical development of Novavax’s COVID-19 vaccine candidate, saying March 10 it agreed to produce the vaccine candidate and had initiated work, anticipating the vaccine candidate will be used in a Phase I study within the next four months. In February, Novavax said it had produced and was assessing multiple nanoparticle vaccine candidates in animal models prior to identifying an optimal candidate for human testing.
References
- ^ “Company Overview of Novavax, Inc”. Bloomberg.com. Archived from the original on 24 February 2017. Retrieved 2 June2019.
- ^ https://www.globenewswire.com/news-release/2021/03/01/2184674/0/en/Novavax-Reports-Fourth-Quarter-and-Full-Year-2020-Financial-Results-and-Operational-Highlights.html
- ^ Jump up to:a b c d e Bell, Jacob (November 14, 2016). “Novavax aims to rebound with restructuring, more trials”. BioPharma Dive. Washington, D.C.: Industry Dive. Archived from the original on 2017-03-29. Retrieved 2017-03-28.
- ^ Thomas, Katie; Twohey, Megan (2020-07-16). “How a Struggling Company Won $1.6 Billion to Make a Coronavirus Vaccine”. The New York Times. ISSN 0362-4331. Retrieved 2021-01-29.
- ^ Taylor, Nick Paul (3 June 2013). “Novavax makes $30M bid for adjuvant business”. FiercePharma. Archived from the original on 14 September 2016. Retrieved 9 September 2016.
- ^ “Gaithersburg Biotech Receives Grant Worth up to $89 million”. Bizjournals.com. Archived from the original on 2017-04-01. Retrieved 2017-03-28.
- ^ “With promising RSV data in hand, Novavax wins $89M Gates grant for PhIII | FierceBiotech”. Fiercebiotech.com. Archivedfrom the original on 2017-04-14. Retrieved 2017-03-28.
- ^ “Novavax RSV vaccine found safe for pregnant women, fetus”. Reuters. 2016-09-29. Archived from the original on 2016-10-07. Retrieved 2017-03-28.
- ^ Herper, Matthew. “Gates Foundation Backs New Shot To Prevent Babies From Dying Of Pneumonia”. Forbes. Archived from the original on 2016-09-21. Retrieved 2017-03-28.
- ^ “Novavax’s Ebola vaccine shows promise in early-stage trial”. Reuters. 2017-07-21. Archived from the original on 2016-10-02. Retrieved 2017-03-28.
- ^ Jump up to:a b c d e f Adams, Ben (September 16, 2016). “Novavax craters after Phase III RSV F vaccine failure; seeks path forward”. FierceBiotech. Questex. Archived from the original on 18 August 2020. Retrieved 25 Jan 2020.
- ^ Shtrubel, Marty (December 12, 2019). “3 Biotech Stocks That Offer the Highest Upside on Wall Street”. Biotech. Nasdaq. Archived from the original on 2020-01-26. Retrieved 25 Jan 2020.
- ^ Jump up to:a b Budwell, George (January 20, 2020). “3 Top Biotech Picks for 2020”. Markets. Nasdaq. Novavax: A catalyst awaits. Archivedfrom the original on 2020-01-25. Retrieved 25 Jan 2020.
- ^ Mark Terry (February 16, 2018). “Why Novavax Could be a Millionaire-Maker Stock”. BioSpace. Archived from the original on 22 November 2020. Retrieved 6 March 2020.
- ^ Jump up to:a b Eric Sagonowsky (2020-05-11). “Novavax scores $384M deal, CEPI’s largest ever, to fund coronavirus vaccine work”. FiercePharma. Archived from the original on 2020-05-16. Retrieved 2020-05-12.
- ^ “Novavax addresses urgent global public health needs with innovative technology”. novavax.com. Archived from the original on 10 September 2020. Retrieved 30 August 2020.
- ^ Sara Gilgore (January 15, 2020). “Novavax earns key FDA status for its flu vaccine. Wall Street took it well”. Washington Business Journal. Archived from the original on 10 November 2020. Retrieved 6 March 2020.
- ^ Sara Gilgore (February 26, 2020). “Novavax is working to advance a potential coronavirus vaccine. So are competitors”. Washington Business Journal. Archived from the original on March 16, 2020. Retrieved March 6, 2020.
- ^ Nidhi Parekh (July 24, 2020). “Novavax: A SARS-CoV-2 Protein Factory to Beat COVID-19”. Archived from the original on November 22, 2020. Retrieved July 24, 2020.
- ^ “Covid-19: Novavax vaccine shows 89% efficacy in UK trials”. BBC news. Retrieved 1 February 2021.
Further reading
- “Novavax, Inc. Common Stock (NVAX) News Headlines”. Market Activity. Nasdaq. Retrieved 25 Jan 2020. Continuously updated listing of Nasdaq publications related to Novavax, newest items first.
External links
- Official website
- Business data for Novavax, Inc.:
General References
Type | Public |
---|---|
Traded as | Nasdaq: NVAX Russell 2000 Component |
Industry | Biotechnology |
Founded | 1987; 34 years ago [1] |
Headquarters | Gaithersburg, Maryland,United States |
Area served | Worldwide |
Key people | Stanley Erck (CEO) |
Products | Vaccines |
Revenue | ![]() |
Number of employees | 500+[3] |
Website | www.novavax.com |
The Novavax COVID-19 vaccine, codenamed NVX-CoV2373, and also called SARS-CoV-2 rS (recombinant spike) protein nanoparticle with Matrix-M1 adjuvant, is a COVID-19 vaccine candidate developed by Novavax and Coalition for Epidemic Preparedness Innovations (CEPI). It requires two doses[1] and is stable at 2 to 8 °C (36 to 46 °F) (refrigerated).[2]
Description
NVX-CoV2373 has been described as both a protein subunit vaccine[3][4][5] and a virus-like particle vaccine,[6][7] though the producers call it a “recombinant nanoparticle vaccine”.[8]
The vaccine is produced by creating an engineered baculovirus containing a gene for a modified SARS-CoV-2 spike protein. The baculovirus then infects a culture of Sf9 moth cells, which create the spike protein and display it on their cell membranes. The spike proteins are then harvested and assembled onto a synthetic lipid nanoparticle about 50 nanometers across, each displaying up to 14 spike proteins.[3][4][8]
The formulation includes a saponin-based adjuvant.[3][4][8]
Development
In January 2020, Novavax announced development of a vaccine candidate, codenamed NVX-CoV2373, to establish immunity to SARS-CoV-2.[9] Novavax’s work is in competition for vaccine development among dozens of other companies.[10]
In March 2020, Novavax announced a collaboration with Emergent BioSolutions for preclinical and early-stage human research on the vaccine candidate.[11] Under the partnership, Emergent BioSolutions will manufacture the vaccine at large scale at their Baltimore facility.[12] Trials have also taken place in the United Kingdom, and subject to regulatory approval, at least 60 million doses will be manufactured by Fujifilm Diosynth Biotechnologies in Billingham for purchase by the UK government.[13][14] They also signed an agreement with Serum Institute of India for mass scale production for developing and low-income countries.[15] It has also been reported, that the vaccine will be manufactured in Spain.[16] The first human safety studies of the candidate, codenamed NVX-CoV2373, started in May 2020 in Australia.[17][18]
In July, the company announced it might receive $1.6 billion from Operation Warp Speed to expedite development of its coronavirus vaccine candidate by 2021—if clinical trials show the vaccine to be effective.[19][20] A spokesperson for Novavax stated that the $1.6 billion was coming from a “collaboration” between the Department of Health and Human Services and Department of Defense,[19][20] where Gen. Gustave F. Perna has been selected as COO for Warp Speed. In late September, Novavax entered the final stages of testing its coronavirus vaccine in the UK. Another large trial was announced to start by October in the US.[21]
In December 2020, Novavax started the PREVENT-19 (NCT04611802) Phase III trial in the US and Mexico.[22][full citation needed][23]
On 28 January 2021, Novavax reported that preliminary results from the United Kingdom trial showed that its vaccine candidate was more than 89% effective.[24][2] However, interim results from a trial in South Africa showed a lower effectiveness rate against the 501.V2 variant of the virus, at around 50-60%.[1][25]
On 12 March 2021, they announced their vaccine candidate was 96.4% effective in preventing the original strain of COVID-19 and 86% effective against the U.K variant. It proved 55% effective against the South African variant in people without HIV/AIDS. It was also 100% effective at preventing severe illness.[citation needed]
Deployment
On 2 February 2021, the Canadian Prime Minister Justin Trudeau announced that Canada has signed a tentative agreement for Novavax to produce millions of doses of its COVID-19 vaccine in Montreal, Canada, once it’s approved for use by Health Canada, making it the first COVID-19 vaccine to be produced domestically.[26]
References
- ^ Jump up to:a b Wadman M, Jon C (28 January 2021). “Novavax vaccine delivers 89% efficacy against COVID-19 in UK—but is less potent in South Africa”. Science. doi:10.1126/science.abg8101.
- ^ Jump up to:a b “New Covid vaccine shows 89% efficacy in UK trials”. BBC News. 28 January 2021. Retrieved 28 January 2021.
- ^ Jump up to:a b c Wadman M (November 2020). “The long shot”. Science. 370 (6517): 649–653. Bibcode:2020Sci…370..649W. doi:10.1126/science.370.6517.649. PMID 33154120.
- ^ Jump up to:a b c Wadman M (28 December 2020). “Novavax launches pivotal U.S. trial of dark horse COVID-19 vaccine after manufacturing delays”. Science. doi:10.1126/science.abg3441.
- ^ Parekh N (24 July 2020). “Novavax: A SARS-CoV-2 Protein Factory to Beat COVID-19”. Archived from the original on 22 November 2020. Retrieved 24 July 2020.
- ^ Chung YH, Beiss V, Fiering SN, Steinmetz NF (October 2020). “COVID-19 Vaccine Frontrunners and Their Nanotechnology Design”. ACS Nano. 14 (10): 12522–12537. doi:10.1021/acsnano.0c07197. PMC 7553041. PMID 33034449.
- ^ Medhi R, Srinoi P, Ngo N, Tran HV, Lee TR (25 September 2020). “Nanoparticle-Based Strategies to Combat COVID-19”. ACS Applied Nano Materials. 3 (9): 8557–8580. doi:10.1021/acsanm.0c01978. PMC 7482545.
- ^ Jump up to:a b c “Urgent global health needs addressed by Novavax”. Novavax. Retrieved 30 January 2021.
- ^ Gilgore S (26 February 2020). “Novavax is working to advance a potential coronavirus vaccine. So are competitors”. Washington Business Journal. Archived from the original on 16 March 2020. Retrieved 6 March 2020.
- ^ “COVID-19 vaccine tracker (click on ‘Vaccines’ tab)”. Milken Institute. 11 May 2020. Archived from the original on 6 June 2020. Retrieved 12 May 2020. Lay summary.
- ^ Gilgore S (10 March 2020). “Novavax’s coronavirus vaccine program is getting some help from Emergent BioSolutions”. Washington Business Journal. Archived from the original on 9 April 2020. Retrieved 10 March 2020.
- ^ McCartney R. “Maryland plays an outsized role in worldwide hunt for a coronavirus vaccine”. Washington Post. Archived from the original on 7 May 2020. Retrieved 8 May 2020.
- ^ Boseley S, Davis N (28 January 2021). “Novavax Covid vaccine shown to be nearly 90% effective in UK trial”. The Guardian. Retrieved 29 January 2021.
- ^ Brown M (14 August 2020). “60m doses of new covid-19 vaccine could be made in Billingham – and be ready for mid-2021”. TeesideLive. Reach. Retrieved 29 January 2021.
- ^ “Novavax signs COVID-19 vaccine supply deal with India’s Serum Institute”. Reuters. 5 August 2020.
- ^ “Spain, again chosen to produce the vaccine to combat COVID-19”. This is the Real Spain. 18 September 2020.
- ^ Sagonowsky E (11 May 2020). “Novavax scores $384M deal, CEPI’s largest ever, to fund coronavirus vaccine work”. FiercePharma. Archived from the original on 16 May 2020. Retrieved 12 May 2020.
- ^ “Novavax starts clinical trial of its coronavirus vaccine candidate”. CNBC. 25 May 2020. Archived from the original on 26 May 2020. Retrieved 26 May 2020.
- ^ Jump up to:a b Thomas K (7 July 2020). “U.S. Will Pay $1.6 Billion to Novavax for Coronavirus Vaccine”. The New York Times. Archived from the original on 7 July 2020. Retrieved 7 July 2020.
- ^ Jump up to:a b Steenhuysen J (7 July 2020). “U.S. government awards Novavax $1.6 billion for coronavirus vaccine”. Reuters. Archived from the original on 14 September 2020. Retrieved 15 September 2020.
- ^ Thomas K, Zimmer C (24 September 2020). “Novavax Enters Final Stage of Coronavirus Vaccine Trials”. The New York Times. ISSN 0362-4331. Archived from the original on 28 September 2020. Retrieved 28 September 2020.
- ^ Clinical trial number NCT04611802 for “A Study Looking at the Efficacy, Immune Response, and Safety of a COVID-19 Vaccine in Adults at Risk for SARS-CoV-2” at ClinicalTrials.gov
- ^ “Phase 3 trial of Novavax investigational COVID-19 vaccine opens”. National Institutes of Health (NIH). 28 December 2020. Retrieved 28 December 2020.
- ^ Lovelace B (28 January 2020). “Novavax says Covid vaccine is more than 89% effective”. CNBC.
- ^ Facher L, Joseph A (28 January 2021). “Novavax says its Covid-19 vaccine is 90% effective in late-stage trial”. Stat. Retrieved 29 January 2021.
- ^ “Canada signs deal to produce Novavax COVID-19 vaccine at Montreal plant”. CP24. 2 February 2021. Retrieved 2 February2021.
Vaccine description | |
---|---|
Target | SARS-CoV-2 |
Vaccine type | Subunit |
Clinical data | |
Other names | NVX-CoV2373 |
Routes of administration | Intramuscular |
ATC code | None |
Identifiers | |
DrugBank | DB15810 |
Part of a series on the |
COVID-19 pandemic |
---|
SARS-CoV-2 (virus)COVID-19 (disease) |
showTimeline |
showLocations |
showInternational response |
showMedical response |
showImpact |
COVID-19 Portal |
vte |
////////////// Novavax, COVID-19, vaccine, CORONA VIRUS, NVX-CoV2373, SARS-CoV-2 rS, TAK 019
#Novavax, #COVID-19, #vaccine, #CORONA VIRUS, #NVX-CoV2373, #SARS-CoV-2 rS, #TAK 019
UPDATE
SARS-CoV-2 Spike glycoprotein vaccine antigen nvx-cov2373
SARS-CoV-2 rS;
Novavax Covid-19 vaccine (TN);
Nuvaxovid (TN)
SARS-CoV-2 rS;
組換えコロナウイルス (SARS-CoV-2) ワクチン;
コロナウイルス(SARS-CoV-2)スパイク糖タンパク質抗原nvx-cov2373ワクチン;
SARS-CoV-2 Spike glycoprotein vaccine antigen nvx-cov2373;
SARS-CoV-2 rS
APPROVED JAPAN Nuvaxovid, 2022/4/19
//////////

AS ON DEC2021 3,491,869 VIEWS ON BLOG WORLDREACH AVAILABLEFOR YOUR ADVERTISEMENT

join me on Linkedin
Anthony Melvin Crasto Ph.D – India | LinkedIn
join me on Researchgate
RESEARCHGATE
join me on Facebook
Anthony Melvin Crasto Dr. | Facebook
join me on twitter
Anthony Melvin Crasto Dr. | twitter
+919321316780 call whatsaapp
EMAIL. amcrasto@amcrasto
/////////////////////////////////////////////////////////////////////////////
/////////SARS-CoV-2 Spike glycoprotein vaccine antigen nvx-cov2373, JAPAN 2022, APPROVALS 2022, VACCINE, COVID 19. CORONA VACCINE, SARS-CoV-2
Tozinameran, Pfizer–BioNTech COVID‑19 vaccine


SEQUENCE1
gagaauaaac uaguauucuu cuggucccca cagacucaga gagaacccgc51caccauguuc guguuccugg ugcugcugcc ucuggugucc agccagugug101ugaaccugac caccagaaca cagcugccuc cagccuacac caacagcuuu151accagaggcg uguacuaccc cgacaaggug uucagaucca gcgugcugca201cucuacccag gaccuguucc ugccuuucuu cagcaacgug accugguucc251acgccaucca cguguccggc accaauggca ccaagagauu cgacaacccc301gugcugcccu ucaacgacgg gguguacuuu gccagcaccg agaaguccaa351caucaucaga ggcuggaucu ucggcaccac acuggacagc aagacccaga401gccugcugau cgugaacaac gccaccaacg uggucaucaa agugugcgag451uuccaguucu gcaacgaccc cuuccugggc gucuacuacc acaagaacaa501caagagcugg auggaaagcg aguuccgggu guacagcagc gccaacaacu551gcaccuucga guacgugucc cagccuuucc ugauggaccu ggaaggcaag601cagggcaacu ucaagaaccu gcgcgaguuc guguuuaaga acaucgacgg651cuacuucaag aucuacagca agcacacccc uaucaaccuc gugcgggauc701ugccucaggg cuucucugcu cuggaacccc ugguggaucu gcccaucggc751aucaacauca cccgguuuca gacacugcug gcccugcaca gaagcuaccu801gacaccuggc gauagcagca gcggauggac agcuggugcc gccgcuuacu851augugggcua ccugcagccu agaaccuucc ugcugaagua caacgagaac901ggcaccauca ccgacgccgu ggauugugcu cuggauccuc ugagcgagac951aaagugcacc cugaaguccu ucaccgugga aaagggcauc uaccagacca1001gcaacuuccg ggugcagccc accgaaucca ucgugcgguu ccccaauauc1051accaaucugu gccccuucgg cgagguguuc aaugccacca gauucgccuc1101uguguacgcc uggaaccgga agcggaucag caauugcgug gccgacuacu1151ccgugcugua caacuccgcc agcuucagca ccuucaagug cuacggcgug1201uccccuacca agcugaacga ccugugcuuc acaaacgugu acgccgacag1251cuucgugauc cggggagaug aagugcggca gauugccccu ggacagacag1301gcaagaucgc cgacuacaac uacaagcugc ccgacgacuu caccggcugu1351gugauugccu ggaacagcaa caaccuggac uccaaagucg gcggcaacua1401caauuaccug uaccggcugu uccggaaguc caaucugaag cccuucgagc1451gggacaucuc caccgagauc uaucaggccg gcagcacccc uuguaacggc1501guggaaggcu ucaacugcua cuucccacug caguccuacg gcuuucagcc1551cacaaauggc gugggcuauc agcccuacag agugguggug cugagcuucg1601aacugcugca ugccccugcc acagugugcg gcccuaagaa aagcaccaau1651cucgugaaga acaaaugcgu gaacuucaac uucaacggcc ugaccggcac1701cggcgugcug acagagagca acaagaaguu ccugccauuc cagcaguuug1751gccgggauau cgccgauacc acagacgccg uuagagaucc ccagacacug1801gaaauccugg acaucacccc uugcagcuuc ggcggagugu cugugaucac1851cccuggcacc aacaccagca aucagguggc agugcuguac caggacguga1901acuguaccga agugcccgug gccauucacg ccgaucagcu gacaccuaca1951uggcgggugu acuccaccgg cagcaaugug uuucagacca gagccggcug2001ucugaucgga gccgagcacg ugaacaauag cuacgagugc gacaucccca2051ucggcgcugg aaucugcgcc agcuaccaga cacagacaaa cagcccucgg2101agagccagaa gcguggccag ccagagcauc auugccuaca caaugucucu2151gggcgccgag aacagcgugg ccuacuccaa caacucuauc gcuaucccca2201ccaacuucac caucagcgug accacagaga uccugccugu guccaugacc2251aagaccagcg uggacugcac cauguacauc ugcggcgauu ccaccgagug2301cuccaaccug cugcugcagu acggcagcuu cugcacccag cugaauagag2351cccugacagg gaucgccgug gaacaggaca agaacaccca agagguguuc2401gcccaaguga agcagaucua caagaccccu ccuaucaagg acuucggcgg2451cuucaauuuc agccagauuc ugcccgaucc uagcaagccc agcaagcgga2501gcuucaucga ggaccugcug uucaacaaag ugacacuggc cgacgccggc2551uucaucaagc aguauggcga uugucugggc gacauugccg ccagggaucu2601gauuugcgcc cagaaguuua acggacugac agugcugccu ccucugcuga2651ccgaugagau gaucgcccag uacacaucug cccugcuggc cggcacaauc2701acaagcggcu ggacauuugg agcaggcgcc gcucugcaga uccccuuugc2751uaugcagaug gccuaccggu ucaacggcau cggagugacc cagaaugugc2801uguacgagaa ccagaagcug aucgccaacc aguucaacag cgccaucggc2851aagauccagg acagccugag cagcacagca agcgcccugg gaaagcugca2901ggacgugguc aaccagaaug cccaggcacu gaacacccug gucaagcagc2951uguccuccaa cuucggcgcc aucagcucug ugcugaacga uauccugagc3001agacuggacc cuccugaggc cgaggugcag aucgacagac ugaucacagg3051cagacugcag agccuccaga cauacgugac ccagcagcug aucagagccg3101ccgagauuag agccucugcc aaucuggccg ccaccaagau gucugagugu3151gugcugggcc agagcaagag aguggacuuu ugcggcaagg gcuaccaccu3201gaugagcuuc ccucagucug ccccucacgg cgugguguuu cugcacguga3251cauaugugcc cgcucaagag aagaauuuca ccaccgcucc agccaucugc3301cacgacggca aagcccacuu uccuagagaa ggcguguucg uguccaacgg3351cacccauugg uucgugacac agcggaacuu cuacgagccc cagaucauca3401ccaccgacaa caccuucgug ucuggcaacu gcgacgucgu gaucggcauu3451gugaacaaua ccguguacga cccucugcag cccgagcugg acagcuucaa3501agaggaacug gacaaguacu uuaagaacca cacaagcccc gacguggacc3551ugggcgauau cagcggaauc aaugccagcg ucgugaacau ccagaaagag3601aucgaccggc ugaacgaggu ggccaagaau cugaacgaga gccugaucga3651ccugcaagaa cuggggaagu acgagcagua caucaagugg cccugguaca3701ucuggcuggg cuuuaucgcc ggacugauug ccaucgugau ggucacaauc3751augcuguguu gcaugaccag cugcuguagc ugccugaagg gcuguuguag3801cuguggcagc ugcugcaagu ucgacgagga cgauucugag cccgugcuga3851agggcgugaa acugcacuac acaugaugac ucgagcuggu acugcaugca3901cgcaaugcua gcugccccuu ucccguccug gguaccccga gucucccccg3951accucggguc ccagguaugc ucccaccucc accugcccca cucaccaccu4001cugcuaguuc cagacaccuc ccaagcacgc agcaaugcag cucaaaacgc4051uuagccuagc cacaccccca cgggaaacag cagugauuaa ccuuuagcaa4101uaaacgaaag uuuaacuaag cuauacuaac cccaggguug gucaauuucg4151ugccagccac acccuggagc uagcaaaaaa aaaaaaaaaa aaaaaaaaaa4201aaaagcauau gacuaaaaaa aaaaaaaaaa aaaaaaaaaa aaaaaaaaaa4251aaaaaaaaaa aaaaaaaaaa aaaaaaaaaa aaaa
Sequence Modifications
Type | Location | Description |
---|---|---|
modified base | g-1 | m7g |
modified base | g-1 | 3′-me |
modified base | a-2 | am |
uncommon link | g-1 – a-2 | 5′->5′ triphosphate |
Tozinameran
Pfizer–BioNTech COVID-19 vaccine
トジナメラン (JAN);
コロナウイルス修飾ウリジンRNAワクチン;
RNA (recombinant 5′-[1,2-[(3′-O-methyl)m7G-(5’→5′)-ppp-Am]]-capped all uridine→N1-methylpseudouridine-substituted severe acute respiratory syndrome coronavirus 2 secretory signal peptide contg. spike glycoprotein S1S2-specifying plus 5′- and 3′-untranslated flanking region-contg. poly(A)-tailed messenger BNT162b2), inner salt
Nucleic Acid Sequence
Sequence Length: 42841106 a 1315 c 1062 g 801 umodified
APPROVED JAPAN Comirnaty, 2021/2/14
CAS 2417899-77-3
Active immunization (SARS-CoV-2) |
Tozinameran is mRNA encoding full length of spike protein analog of SARS-CoV-2
Target Severe acute respiratory syndrome coronavirus 2 spike glycoprotein
Coronavirus disease – COVID-19
FORM | ROUTE | STRENGTH |
---|---|---|
Injection, suspension | Intramuscular | 0.23 mg/1.8mL |
Suspension | Intramuscular | 30 mcg |
NAME | INGREDIENTS | DOSAGE | ROUTE | LABELLER | MARKETING START | MARKETING END | ||
---|---|---|---|---|---|---|---|---|
Pfizer-BioNTech Covid-19 Vaccine | Pfizer-BioNTech Covid-19 Vaccine (0.23 mg/1.8mL) | Injection, suspension | Intramuscular | Pfizer Manufacturing Belgium NV | 2020-12-12 | Not applicable |
NAME | DOSAGE | STRENGTH | ROUTE | LABELLER | MARKETING START | MARKETING END | ||
---|---|---|---|---|---|---|---|---|
Comirnaty | 30 mcg | Intramuscular | Bio N Tech Manufacturing Gmb H | 2021-01-06 | Not applicable | |||
Pfizer-BioNTech Covid-19 Vaccine | Suspension | 30 mcg | Intramuscular | Biontech Manufacturing Gmbh | 2020-12-14 | Not applicable | ||
Pfizer-BioNTech Covid-19 Vaccine | Injection, suspension | 0.23 mg/1.8mL | Intramuscular | Pfizer Manufacturing Belgium NV | 2020-12-12 | Not applicable |
The Pfizer–BioNTech COVID‑19 vaccine (pINN: tozinameran), sold under the brand name Comirnaty,[13] is a COVID-19 vaccine developed by the German company BioNTech in cooperation with Pfizer. It is both the first COVID-19 vaccine to be authorized by a stringent regulatory authority for emergency use[14][15] and the first cleared for regular use.[16]
It is given by intramuscular injection. It is an RNA vaccine composed of nucleoside-modified mRNA (modRNA) encoding a mutated form of the spike protein of SARS-CoV-2, which is encapsulated in lipid nanoparticles.[17] The vaccination requires two doses given three weeks apart.[18][19][20] Its ability to prevent severe infection in children, pregnant women, or immunocompromised people is unknown, as is the duration of the immune effect it confers.[20][21][22] As of February 2021, it is one of two RNA vaccines being deployed against COVID‑19, the other being the Moderna COVID‑19 vaccine. A third mRNA-based COVID-19 vaccine, CVnCoV, is in late-stage testing.[23]
Trials began in April 2020; by November, the vaccine had been tested on more than 40,000 people.[24] An interim analysis of study data showed a potential efficacy of over 90% in preventing infection within seven days of a second dose.[19][20] The most common side effects include mild to moderate pain at the injection site, fatigue, and headache.[25][26] As of December 2020, reports of serious side effects, such as allergic reactions, have been very rare,[a] and no long-term complications have been reported.[28] Phase III clinical trials are ongoing: monitoring of the primary outcomes will continue until August 2021, while monitoring of the secondary outcomes will continue until January 2023.[18]
In December 2020, the United Kingdom was the first country to authorize the vaccine on an emergency basis,[28] soon followed by the United States, the European Union and several other countries globally.[29][30][6][31][32]
BioNTech is the initial developer of the vaccine, and partnered with Pfizer for development, clinical research, overseeing the clinical trials, logistics, finances and for manufacturing worldwide with the exception of China.[33] The license to distribute and manufacture in China was purchased by Fosun, alongside its investment in BioNTech.[34][35] Distribution in Germany and Turkey is by BioNTech itself.[36] Pfizer indicated in November 2020, that 50 million doses could be available globally by the end of 2020, with about 1.3 billion doses in 2021.[20]
Pfizer has advanced purchase agreements of about US$3 billion for providing a licensed vaccine in the United States, the European Union, the United Kingdom, Japan, Canada, Peru, Singapore, and Mexico.[37][38] Distribution and storage of the vaccine is a logistics challenge because it needs to be stored at temperatures between −80 and −60 °C (−112 and −76 °F),[39] until five days before vaccination[38][39] when it can be stored at 2 to 8 °C (36 to 46 °F), and up to two hours at temperatures up to 25 °C (77 °F)[40][11] or 30 °C (86 °F).[41][42] In February 2021, Pfizer and BioNTech asked the U.S. Food and Drug Administration (FDA) to update the emergency use authorization (EUA) to permit the vaccine to be stored at between −25 and −15 °C (−13 and 5 °F) for up to two weeks before use.[43]
Development and funding
Before COVID-19 vaccines, a vaccine for an infectious disease had never before been produced in less than several years, and no vaccine existed for preventing a coronavirus infection in humans.[44] After the COVID-19 virus was detected in December 2019,[45] the development of BNT162b2 was initiated on 10 January 2020, when the SARS-CoV-2 genetic sequences were released by the Chinese Center for Disease Control and Prevention via GISAID,[46][47][48] triggering an urgent international response to prepare for an outbreak and hasten development of preventive vaccines.[49][50]
In January 2020, German biotech-company BioNTech started its program ‘Project Lightspeed’ to develop a vaccine against the new COVID‑19 virus based on its already established mRNA-technology.[24] Several variants of the vaccine were created in their laboratories in Mainz, and 20 of those were presented to experts of the Paul-Ehrlich-Institute in Langen.[51] Phase I / II Trials were started in Germany on 23 April 2020, and in the U.S. on 4 May 2020, with four vaccine candidates entering clinical testing. The Initial Pivotal Phase II / III Trial with the lead vaccine candidate ‘BNT162b2’ began in July. The Phase III results indicating a 95% effectiveness of the developed vaccine were published on 18 November 2020.[24]
BioNTech received a US$135 million investment from Fosun in March 2020, in exchange for 1.58 million shares in BioNTech and the future development and marketing rights of BNT162b2 in China,[35] Hong Kong, Macau and Taiwan.[52]
In June 2020, BioNTech received €100 million (US$119 million) in financing from the European Commission and European Investment Bank.[53] In September 2020, the German government granted BioNTech €375 million (US$445 million) for its COVID‑19 vaccine development program.[54]
Pfizer CEO Albert Bourla stated that he decided against taking funding from the US government’s Operation Warp Speed for the development of the vaccine “because I wanted to liberate our scientists [from] any bureaucracy that comes with having to give reports and agree how we are going to spend the money in parallel or together, etc.” Pfizer did enter into an agreement with the US for the eventual distribution of the vaccine, as with other countries.[55]
Clinical trials
See also: COVID-19 vaccine § Clinical trials started in 2020
Preliminary results from Phase I–II clinical trials on BNT162b2, published in October 2020, indicated potential for its efficacy and safety.[17][56] During the same month, the European Medicines Agency (EMA) began a periodic review of BNT162b2.[57]
The study of BNT162b2 is a continuous-phase trial in Phase III as of November 2020.[18] It is a “randomized, placebo-controlled, observer-blind, dose-finding, vaccine candidate-selection, and efficacy study in healthy individuals”.[18] The early-stage research determined the safety and dose level for two vaccine candidates, with the trial expanding during mid-2020 to assess efficacy and safety of BNT162b2 in greater numbers of participants, reaching tens of thousands of people receiving test vaccinations in multiple countries in collaboration with Pfizer and Fosun.[20][35]
The Phase III trial assesses the safety, efficacy, tolerability, and immunogenicity of BNT162b2 at a mid-dose level (two injections separated by 21 days) in three age groups: 12–15 years, 16–55 years or above 55 years.[18] For approval in the EU, an overall vaccine efficacy of 95% was confirmed by the EMA.[58] The EMA clarified that the second dose should be administered three weeks after the first dose.[59]
Efficacy endpoint | Vaccine efficacy (95% confidence interval) [%] |
---|---|
After dose 1 to before dose 2 | 52.4 (29.5, 68.4) |
≥10 days after dose 1 to before dose 2 | 86.7 (68.6, 95.4) |
Dose 2 to 7 days after dose 2 | 90.5 (61.0, 98.9) |
≥7 days after dose 2 (subjects without evidence of infection prior to 7 days after dose 2) | |
Overall | 95.0 (90.0, 97.9) |
16–55 years | 95.6 (89.4, 98.6) |
≥55 years | 93.7 (80.6, 98.8) |
≥65 years | 94.7 (66.7, 99.9) |
The ongoing Phase III trial, which is scheduled to run from 2020 to 2022, is designed to assess the ability of BNT162b2 to prevent severe infection, as well as the duration of immune effect.[20][21][22]
Pfizer and BioNTech started a Phase II/III randomized control trial in healthy pregnant women 18 years of age and older (NCT04754594).[60] The study will evaluate 30 µg of BNT162b2 or placebo administered via intramuscular injection in 2 doses, 21 days apart. The Phase II portion of the study will include approximately 350 pregnant women randomized 1:1 to receive BNT162b2 or placebo at 27 to 34 weeks’ gestation. The Phase III portion of this study will assess the safety, tolerability, and immunogenicity of BNT162b2 or placebo among pregnant women enrolled at 24 to 34 weeks’ gestation. Pfizer and BioNTech announced on 18 February 2021 that the first participants received their first dose in this trial.[61]
Vaccine technology
See also: RNA vaccine and COVID-19 vaccine § Technology platforms
The BioNTech technology for the BNT162b2 vaccine is based on use of nucleoside-modified mRNA (modRNA) which encodes part of the spike protein found on the surface of the SARS-CoV-2 coronavirus (COVID‑19), triggering an immune response against infection by the virus protein.[62]
The vaccine candidate BNT162b2 was chosen as the most promising among three others with similar technology developed by BioNTech.[18][62][56] Prior to choosing BNT162b2, BioNTech and Pfizer had conducted Phase I trials on BNT162b1 in Germany and the United States, while Fosun performed a Phase I trial in China.[17][63] In these Phase I studies, BNT162b2 was shown to have a better safety profile than the other three BioNTech candidates.[63]
Sequence
The modRNA sequence of the vaccine is 4,284 nucleotides long.[64] It consists of a five-prime cap; a five prime untranslated region derived from the sequence of human alpha globin; a signal peptide (bases 55–102) and two proline substitutions (K986P and V987P, designated “2P”) that cause the spike to adopt a prefusion-stabilized conformation reducing the membrane fusion ability, increasing expression and stimulating neutralizing antibodies;[17][65] a codon-optimized gene of the full-length spike protein of SARS-CoV-2 (bases 103–3879); followed by a three prime untranslated region (bases 3880–4174) combined from AES and mtRNR1 selected for increased protein expression and mRNA stability[66] and a poly(A) tail comprising 30 adenosine residues, a 10-nucleotide linker sequence, and 70 other adenosine residues (bases 4175–4284).[64] The sequence contains no uridine residues; they are replaced by 1-methyl-3′-pseudouridylyl.[64]
Composition
In addition to the mRNA molecule, the vaccine contains the following inactive ingredients (excipients):[67][68][8]
- ALC-0315, ((4-hydroxybutyl)azanediyl)bis(hexane-6,1-diyl)bis(2-hexyldecanoate)
- ALC-0159, 2-[(polyethylene glycol)-2000]-N,N-ditetradecylacetamide
- 1,2-distearoyl-sn-glycero-3-phosphocholine (DSPC)
- cholesterol
- dibasic sodium phosphate dihydrate
- monobasic potassium phosphate
- potassium chloride
- sodium chloride
- sucrose
- water for injection
The first four of these are lipids. The lipids and modRNA together form nanoparticles. ALC-0159 is a polyethylene glycol conjugate (that is, a PEGylated lipid).[69]
The vaccine is supplied in a multidose vial as “a white to off-white, sterile, preservative-free, frozen suspension for intramuscular injection“.[11][12] It must be thawed to room temperature and diluted with normal saline before administration.[12]
Authorizations
Expedited
The United Kingdom’s Medicines and Healthcare products Regulatory Agency (MHRA) gave the vaccine “rapid temporary regulatory approval to address significant public health issues such as a pandemic” on 2 December 2020, which it is permitted to do under the Medicines Act 1968.[70] It was the first COVID‑19 vaccine to be approved for national use after undergoing large scale trials,[71] and the first mRNA vaccine to be authorized for use in humans.[14][72] The United Kingdom thus became the first Western country to approve a COVID‑19 vaccine for national use,[73] although the decision to fast-track the vaccine was criticised by some experts.[74]
On 8 December 2020, Margaret “Maggie” Keenan, 90, from Fermanagh, became the first person to receive the vaccine.[75] In a notable example of museums documenting the pandemic, the vial and syringe used for that first dose were saved acquired by The Science Museum in London for its permanent collection.[76] By 20 December, 521,594 UK residents had received the vaccine as part of the national vaccination programme. 70% had been to people aged 80 or over.[77]
After the United Kingdom, the following countries expedited processes to approve the Pfizer–BioNTech COVID‑19 vaccine for use: Argentina,[78] Australia,[79] Bahrain,[80] Canada,[7][81] Chile,[82] Costa Rica,[83] Ecuador,[82] Hong Kong,[84] Iraq,[85] Israel,[86] Jordan,[87] Kuwait,[88] Mexico,[89] Oman,[90] Panama,[91] the Philippines,[92] Qatar,[93] Saudi Arabia,[32][94] Singapore,[95][96] the United Arab Emirates,[97] and the United States.[10]
The World Health Organization (WHO) authorized it for emergency use.[98]
In the United States, an emergency use authorization (EUA) is “a mechanism to facilitate the availability and use of medical countermeasures, including vaccines, during public health emergencies, such as the current COVID‑19 pandemic”, according to the FDA.[99] Following an EUA issuance, BioNTech and Pfizer are expected to continue the Phase III clinical trial to finalize safety and efficacy data, leading to application for licensure (approval) of the vaccine in the United States.[99][100][101] The United States Centers for Disease Control and Prevention (CDC) Advisory Committee on Immunization Practices (ACIP) approved recommendations for vaccination of those aged 16 years or older.[102][103]
Standard
On 19 December 2020, the Swiss Agency for Therapeutic Products (Swissmedic) approved the Pfizer–BioNTech COVID‑19 vaccine for regular use, two months after receiving the application, stating that the vaccine fully complied with the requirements of safety, efficacy and quality. This is the first authorization under a standard procedure.[1][104] On 23 December, a Lucerne resident, a 90-year-old woman, became the first person to receive the vaccine in Switzerland.[105] This marked the beginning of mass vaccination in continental Europe.[106]
On 21 December 2020, the Committee for Medicinal Products for Human Use (CHMP) of the European Medicines Agency (EMA) recommended granting conditional marketing authorization for the Pfizer–BioNTech COVID‑19 vaccine under the brand name Comirnaty.[2][107][108] The recommendation was accepted by the European Commission the same day.[107][109]
On February 23, 2021, the Brazilian Health Regulatory Agency approved the Pfizer–BioNTech COVID-19 vaccine under its standard marketing authorization procedure. It became the first COVID-19 vaccine to receive definitive registration rather than emergency use authorization in the country.[110]
Adverse effects
The adverse effect profile of the Pfizer–BioNTech COVID‑19 vaccine is similar to that of other adult vaccines.[20] During clinical trials, the side effects deemed very common[a] are (in order of frequency): pain and swelling at the injection site, tiredness, headache, muscle aches, chills, joint pain, and fever.[68] Fever is more common after the second dose.[68] These effects are predictable and to be expected, and it is particularly important that people be aware of this to prevent vaccine hesitancy.[111]
Severe allergic reaction has been observed in approximately 11 cases per million doses of vaccine administered.[112][113] According to a report by the US Centers for Disease Control and Prevention 71% of those allergic reactions happened within 15 minutes of vaccination and mostly (81%) among people with a documented history of allergies or allergic reactions.[112] The UK’s Medicines and Healthcare products Regulatory Agency (MHRA) advised on 9 December 2020, that people who have a history of “significant” allergic reaction should not receive the Pfizer–BioNTech COVID‑19 vaccine.[114][115][116] On 12 December, the Canadian regulator followed suit, noting that: “Both individuals in the U.K. had a history of severe allergic reactions and carried adrenaline auto injectors. They both were treated and have recovered.”[67]
On 28 January 2021, the European Union published a COVID-19 vaccine safety update which found that “the benefits of Comirnaty in preventing COVID‑19 continue to outweigh any risks, and there are no recommended changes regarding the use the vaccine.”[113][117] No new side effects were identified.[113]
Manufacturing

A doctor holding the Pfizer vaccine
Pfizer and BioNTech are manufacturing the vaccine in their own facilities in the United States and in Europe in a three-stage process. The first stage involves the molecular cloning of DNA plasmids that code for the spike protein by infusing them into Escherichia coli bacteria. In the United States, this stage is conducted at a small pilot plant in Chesterfield, Missouri[118] (near St. Louis). After four days of growth, the bacteria are killed and broken open, and the contents of their cells are purified over a week and a half to recover the desired DNA product. The DNA is stored in tiny bottles and frozen for shipment. Safely and quickly transporting the DNA at this stage is so important that Pfizer has used its company jet and helicopter to assist.[119]
The second stage is being conducted at plants in Andover, Massachusetts[120] in the United States, and in Germany. The DNA is used as a template to build the desired mRNA strands. Once the mRNA has been created and purified, it is frozen in plastic bags about the size of a large shopping bag, of which each can hold up to 5 to 10 million doses. The bags are placed on special racks on trucks which take them to the next plant.[119]
The third stage is being conducted at plants in Portage, Michigan[121] (near Kalamazoo) in the United States, and Puurs in Belgium. This stage involves combining the mRNA with lipid nanoparticles, then filling vials, boxing vials, and freezing them.[119] Croda International subsidiary Avanti Polar Lipids is providing the requisite lipids.[122] As of November 2020, the major bottleneck in the manufacturing process was combining mRNA with lipid nanoparticles.[119]
In February 2021, Pfizer revealed this entire sequence initially took about 110 days on average from start to finish, and that the company was making progress on reducing that number to 60 days.[123] Vaccine manufacturers normally take several years to optimize the process of making a particular vaccine for speed and cost-effectiveness before attempting large-scale production.[123] Due to the urgency presented by the COVID-19 pandemic, Pfizer began production immediately with the process by which the vaccine had been originally formulated in the laboratory, then started to identify ways to safely speed up and scale up that process.[123]
BioNTech announced in September 2020 that it had signed an agreement to acquire from Novartis a manufacturing facility in Marburg, Germany, to expand their vaccine production capacity.[124] Once fully operational, the facility would produce up to 750 million doses per year, or over 60 million doses per month. The site will be the third BioNTech facility in Europe which currently produces the vaccine, while Pfizer operates at least four production sites in the United States and Europe.
Advance orders and logistics
Pfizer indicated in its 9 November press release that 50 million doses could be available by the end of 2020, with about 1.3 billion doses provided globally by 2021.[20] In February 2021, BioNTech announced it would increase production by more than 50% to manufacture two billion doses in 2021.[125]
In July 2020, the vaccine development program Operation Warp Speed placed an advance order of US$1.95 billion with Pfizer to manufacture 100 million doses of a COVID‑19 vaccine for use in the United States if the vaccine was shown to be safe and effective.[34][126][127][128] By mid-December 2020, Pfizer had agreements to supply 300 million doses to the European Union,[129] 120 million doses to Japan,[130] 40 million doses (10 million before 2021) to the United Kingdom,[22] 20 million doses to Canada,[131] an unspecified number of doses to Singapore,[132] and 34.4 million doses to Mexico.[133] Fosun also has agreements to supply 10 million doses to Hong Kong and Macau.[134] The Hong Kong government said it would receive its first batch of one million doses by the first quarter of 2021.[135]
BioNTech and Fosun agreed to supply Mainland China with a batch of 100 million doses in 2021, subject to regulatory approval. The initial supply will be delivered from BioNTech’s production facilities in Germany.[136]
The vaccine is being delivered in vials that, once diluted, contain 2.25 ml of vaccine (0.45 ml frozen plus 1.8ml diluent).[101] According to the vial labels, each vial contains five 0.3 ml doses, however excess vaccine may be used for one, or possibly two, additional doses.[101][137] The use of low dead space syringes to obtain the additional doses is preferable, and partial doses within a vial should be discarded.[101][138] The Italian Medicines Agency officially authorized the use of excess doses remaining within single vials.[139] As of 8 January 2021, each vial contains six doses.[68][140][141][138] In the United States, vials will be counted as five doses when accompanied by regular syringes and as six doses when accompanied by low dead space syringes.[142]

Temperature the Pfizer vaccine must be kept at to ensure effectiveness, roughly between −80 and −60 °C (−112 and −76 °F)
Logistics in developing countries which have preorder agreements with Pfizer—such as Ecuador and Peru—remain unclear.[38] Even high-income countries have limited cold chain capacity for ultracold transport and storage of a vaccine that degrades within five days when thawed, and requires two shots three weeks apart.[38] The vaccine needs to be stored and transported at ultracold temperatures between −80 and −60 °C (−112 and −76 °F),[39][22][38][143][144] much lower than for the similar Moderna vaccine. The head of Indonesia‘s Bio Farma Honesti Basyir stated that purchasing the vaccine is out of the question for the world’s fourth-most populous country, given that it did not have the necessary cold chain capability. Similarly, India’s existing cold chain network can only handle temperatures between 2 and 8 °C (36 and 46 °F), far above the requirements of the vaccine.[145][146]
In January 2021, Pfizer and BioNTech offered to supply 50 million doses of COVID‑19 vaccine for health workers across Africa between March and the end of 2021, at a discounted price of US$10 per dose.[147]
Name
BNT162b2 was the code name during development and testing,[17][148] tozinameran is the proposed international nonproprietary name (pINN),[149] and Comirnaty is the brand name.[1][2] According to BioNTech, the name Comirnaty “represents a combination of the terms COVID‑19, mRNA, community, and immunity.”[150][151]
The vaccine also has the common name “COVID‑19 mRNA vaccine (nucleoside-modified)”[2] and may be distributed in packaging with the name Pfizer–BioNTech COVID‑19 Vaccine.”[152]
How the Pfizer-BioNTech Vaccine Works
By Jonathan Corum and Carl ZimmerUpdated Jan. 21, 2021Leer en español

The German company BioNTech partnered with Pfizer to develop and test a coronavirus vaccine known as BNT162b2, the generic name tozinameran or the brand name Comirnaty. A clinical trial demonstrated that the vaccine has an efficacy rate of 95 percent in preventing Covid-19.
A Piece of the Coronavirus
The SARS-CoV-2 virus is studded with proteins that it uses to enter human cells. These so-called spike proteins make a tempting target for potential vaccines and treatments.

Spikes
Spike
protein
gene
CORONAVIRUS
Like the Moderna vaccine, the Pfizer-BioNTech vaccine is based on the virus’s genetic instructions for building the spike protein.
mRNA Inside an Oily Shell
The vaccine uses messenger RNA, genetic material that our cells read to make proteins. The molecule — called mRNA for short — is fragile and would be chopped to pieces by our natural enzymes if it were injected directly into the body. To protect their vaccine, Pfizer and BioNTech wrap the mRNA in oily bubbles made of lipid nanoparticles.

Lipid nanoparticles
surrounding mRNA
Because of their fragility, the mRNA molecules will quickly fall apart at room temperature. Pfizer is building special containers with dry ice, thermal sensors and GPS trackers to ensure the vaccines can be transported at –94°F (–70°C) to stay viable.
Entering a Cell
After injection, the vaccine particles bump into cells and fuse to them, releasing mRNA. The cell’s molecules read its sequence and build spike proteins. The mRNA from the vaccine is eventually destroyed by the cell, leaving no permanent trace.

VACCINE
PARTICLES
VACCINATED
CELL
Spike
protein
mRNA
Translating mRNA
Three spike
proteins combine
Spike
Cell
nucleus
Spikes
and protein
fragments
Displaying
spike protein
fragments
Protruding
spikes
Some of the spike proteins form spikes that migrate to the surface of the cell and stick out their tips. The vaccinated cells also break up some of the proteins into fragments, which they present on their surface. These protruding spikes and spike protein fragments can then be recognized by the immune system.
Spotting the Intruder
When a vaccinated cell dies, the debris will contain many spike proteins and protein fragments, which can then be taken up by a type of immune cell called an antigen-presenting cell.

Debris from
a dead cell
Engulfing
a spike
ANTIGEN-
PRESENTING
CELL
Digesting
the proteins
Presenting a
spike protein
fragment
HELPER
T CELL
The cell presents fragments of the spike protein on its surface. When other cells called helper T cells detect these fragments, the helper T cells can raise the alarm and help marshal other immune cells to fight the infection.
Making Antibodies
Other immune cells, called B cells, may bump into the coronavirus spikes on the surface of vaccinated cells, or free-floating spike protein fragments. A few of the B cells may be able to lock onto the spike proteins. If these B cells are then activated by helper T cells, they will start to proliferate and pour out antibodies that target the spike protein.

HELPER
T CELL
Activating
the B cell
Matching
surface proteins
VACCINATED
CELL
B CELL
SECRETED
ANTIBODIES
Stopping the Virus
The antibodies can latch onto coronavirus spikes, mark the virus for destruction and prevent infection by blocking the spikes from attaching to other cells.

ANTIBODIES
VIRUS
Killing Infected Cells
The antigen-presenting cells can also activate another type of immune cell called a killer T cell to seek out and destroy any coronavirus-infected cells that display the spike protein fragments on their surfaces.

ANTIGEN-PRESENTING CELL Presenting a spike protein fragment ACTIVATED KILLER T CELL INFECTED CELL Beginning to kill the infected cell
Remembering the Virus
The Pfizer-BioNTech vaccine requires two injections, given 21 days apart, to prime the immune system well enough to fight off the coronavirus. But because the vaccine is so new, researchers don’t know how long its protection might last.

First dose, 0.3ml
Second dose, 21 days later
A preliminary study found that the vaccine seems to offer strong protection about 10 days after the first dose, compared with people taking a placebo:

Cumulative incidence of Covid-19 among clinical trial participants 2.5% 2.0 People taking a placebo
1.5 1.0 Second dose First dose People taking the
Pfizer-BioNTech vaccine
0.5
0
1
2
3
4
8
12
16
Weeks after the first dose
It’s possible that in the months after vaccination, the number of antibodies and killer T cells will drop. But the immune system also contains special cells called memory B cells and memory T cells that might retain information about the coronavirus for years or even decades.
For more about the vaccine, see Pfizer’s Covid Vaccine: 11 Things You Need to Know.
Preparation and Injection
Each vial of the vaccine contains 5 doses of 0.3 milliliters. The vaccine must be thawed before injection and diluted with saline. After dilution the vial must be used within six hours.

A diluted vial of the vaccine at Royal Free Hospital in London.Jack Hill/Agence France-Presse
References
- ^ Jump up to:a b According to the British National Formulary and MedDRA conventions, side effects are “very common” when they occur in more than 1 in 10 instances; “common”, 1 in 100 to 1 in 10; “uncommon”, 1 in 1,000 to 1 in 100; “rare”, 1 in 10,000 to 1 in 1,000; and “very rare” when they occur in less than 1 in 10,000 instances.[27]
- ^ Jump up to:a b c d “Swissmedic grants authorisation for the first COVID-19 vaccine in Switzerland”(Press release). Swiss Agency for Therapeutic Products (Swissmedic). 19 December 2020. Retrieved 19 December 2020.
- ^ Jump up to:a b c d e “Comirnaty EPAR”. European Medicines Agency (EMA). Retrieved 23 December 2020.
- ^ “Comirnaty”. Therapeutic Goods Administration (TGA). Retrieved 25 January 2021.
- ^ “Comirnaty (BNT162b2 [mRNA]) COVID‑19 Vaccine Product Information” (PDF). Therapeutic Goods Administration (TGA). Retrieved 25 January 2021.
- ^ Australian Public Assessment Report for BNT162b2 (mRNA) (PDF) (Report). Therapeutic Goods Administration (TGA). Retrieved 25 January 2021.
- ^ Jump up to:a b “Regulatory Decision Summary – Pfizer-BioNTech COVID-19 Vaccine”. Health Canada. 9 December 2020. Archived from the original on 9 December 2020. Retrieved 9 December 2020.
- ^ Jump up to:a b “Pfizer-BioNTech COVID-19 Vaccine (tozinameran)”. Health Canada. Retrieved 15 December 2020.
- ^ Jump up to:a b “Information for Healthcare Professionals on Pfizer/BioNTech COVID-19 vaccine”. Medicines and Healthcare products Regulatory Agency (MHRA). 10 December 2020. Retrieved 21 December 2020.
- ^ “Conditions of Authorisation for Pfizer/BioNTech COVID-19 vaccine”. Medicines and Healthcare products Regulatory Agency (MHRA). 31 December 2020. Retrieved 8 January2021.
- ^ Jump up to:a b “FDA Takes Key Action in Fight Against COVID-19 By Issuing Emergency Use Authorization for First COVID-19 Vaccine” (Press release). U.S. Food and Drug Administration (FDA). 11 December 2020. Retrieved 11 December 2020.
This article incorporates text from this source, which is in the public domain.
- ^ Jump up to:a b c “Pfizer-BioNTech COVID-19 Vaccine- rna ingredient bnt-162b2 injection, suspension”. DailyMed. Retrieved 14 December 2020.
- ^ Jump up to:a b c Pfizer-BioNTech COVID-19 Vaccine Emergency Use Authorization Review Memorandum (PDF). U.S. Food and Drug Administration (FDA) (Report). 14 December 2020. Retrieved 14 December 2020.
This article incorporates text from this source, which is in the public domain.
- ^ “Comirnaty EPAR”. European Medicines Agency (EMA). Retrieved 23 December 2020.
- ^ Jump up to:a b “UK medicines regulator gives approval for first UK COVID-19 vaccine” (Press release). Medicines and Healthcare products Regulatory Agency (MHRA). 2 December 2020. Retrieved 2 December 2020.
- ^ Boseley S, Halliday J (2 December 2020). “UK approves Pfizer/BioNTech Covid vaccine for rollout next week”. The Guardian. Retrieved 14 December 2020.
- ^ “Swissmedic grants authorisation for the first COVID-19 vaccine in Switzerland” (Press release). Swiss Agency for Therapeutic Products (Swissmedic). 19 December 2020. Retrieved 19 December 2020.
- ^ Jump up to:a b c d e Walsh EE, Frenck RW, Falsey AR, Kitchin N, Absalon J, Gurtman A, et al. (October 2020). “Safety and Immunogenicity of Two RNA-Based Covid-19 Vaccine Candidates”. The New England Journal of Medicine. 383 (25): 2439–50. doi:10.1056/NEJMoa2027906. PMC 7583697. PMID 33053279.
- ^ Jump up to:a b c d e f Clinical trial number NCT04368728 for “NCT04368728: Study to Describe the Safety, Tolerability, Immunogenicity, and Efficacy of RNA Vaccine Candidates Against COVID-19 in Healthy Individuals” at ClinicalTrials.gov
- ^ Jump up to:a b Palca J (9 November 2020). “Pfizer says experimental COVID-19 vaccine is more than 90% effective”. NPR. Archived from the original on 9 November 2020. Retrieved 9 November 2020.
- ^ Jump up to:a b c d e f g h Herper M (9 November 2020). “Covid-19 vaccine from Pfizer and BioNTech is strongly effective, early data from large trial indicate”. STAT. Archived from the original on 9 November 2020. Retrieved 9 November 2020.
- ^ Jump up to:a b Edwards E (9 November 2020). “Pfizer’s Covid-19 vaccine promising, but many questions remain”. NBC News. Archived from the original on 22 November 2020. Retrieved 12 November 2020.
- ^ Jump up to:a b c d Gallagher J (9 November 2020). “Covid vaccine: First ‘milestone’ vaccine offers 90% protection”. BBC News. Archived from the original on 26 November 2020. Retrieved 9 November 2020.
- ^ “CureVac Initiates Rolling Submission With European Medicines Agency for COVID-19 Vaccine Candidate, CVnCoV”. CureVac (Press release).
- ^ Jump up to:a b c “Update on our COVID-19 vaccine development program with BNT162b2” (PDF)(Press release). BioNTech. 2 December 2020. Retrieved 12 December 2020.
- ^ Polack FP, Thomas SJ, Kitchin N, Absalon J, Gurtman A, Lockhart S, et al. (December 2020). “Safety and Efficacy of the BNT162b2 mRNA Covid-19 Vaccine”. N Engl J Med. 383 (27): 2603–2615. doi:10.1056/NEJMoa2034577. PMC 7745181. PMID 33301246.
- ^ “Questions and Answers About Pfizer-BioNTech COVID-19 Vaccine”. Pfizer. Retrieved 16 December 2020.
- ^ “Adverse reactions to drugs”. British National Formulary. Retrieved 19 December 2020.
- ^ Jump up to:a b “Coronavirus vaccine”. National Health Service. 7 December 2020. Archived from the original on 7 December 2020. Retrieved 7 December 2020.
- ^ Commissioner, Office of the (3 February 2021). “Pfizer-BioNTech COVID-19 Vaccine”. FDA.
- ^ “EMA recommends first COVID-19 vaccine for authorisation in the EU”. European Medicines Agency.
- ^ “Bahrain becomes second country to approve Pfizer COVID-19 vaccine”. Al Jazeera. Archived from the original on 4 December 2020. Retrieved 5 December 2020.
* “Coronavirus: Saudi Arabia approves Pfizer COVID-19 vaccine for use”. Al Arabiya English. 10 December 2020. Archived from the original on 11 December 2020. Retrieved 10 December 2020.
* Solomon DB, Torres N (11 December 2020). “Mexico approves emergency use of Pfizer’s COVID-19 vaccine”. Reuters. Retrieved 12 December 2020.
* Thomas K (20 November 2020). “F.D.A. Clears Pfizer Vaccine, and Millions of Doses Will Be Shipped Right Away”. The New York Times. Archived from the original on 12 December 2020. Retrieved 11 December 2020.
* “First shipments of Pfizer-BioNTech vaccine in Singapore by end-Dec; enough vaccines for all by Q3 2021”. The Straits Times. 14 December 2020. Retrieved 14 December 2020. - ^ Jump up to:a b Al Mulla Y (13 December 2020). “Kuwait approves emergency use of Pfizer vaccine”. Gulf News. Retrieved 14 December 2020.
- ^ Browne R (11 November 2020). “What you need to know about BioNTech – the European company behind Pfizer’s Covid-19 vaccine”. CNBC. Retrieved 14 January 2021.
- ^ Jump up to:a b Thomas K, Gelles D, Zimmer C (9 November 2020). “Pfizer’s early data shows vaccine is more than 90% effective”. The New York Times. Archived from the original on 23 November 2020. Retrieved 9 November 2020.
- ^ Jump up to:a b c Burger L (15 March 2020). “BioNTech in China alliance with Fosun over coronavirus vaccine candidate”. Reuters. Archived from the original on 14 November 2020. Retrieved 10 November 2020.
- ^ “Pfizer and BioNTech Celebrate Historic First Authorization in the U.S. of Vaccine to Prevent COVID-19”. Pfizer Inc. and BioNTech SE.
- ^ “Securing Singapore’s access to COVID-19 vaccines”. gov.sg. Government of Singapore. 14 December 2020. Retrieved 1 February 2021.
- ^ Jump up to:a b c d e “Deep-freeze hurdle makes Pfizer’s vaccine one for the rich”. Bloomberg. 10 November 2020. Archived from the original on 22 November 2020. Retrieved 12 November 2020.
Vaccine goes bad five days after thawing, requires two shots; Many nations face costly ramp up of cold-chain infrastructure
- ^ Jump up to:a b c “Pfizer-BioNTech COVID-19 Vaccine Vaccination Storage & Dry Ice Safety Handling”. Pfizer. Retrieved 17 December 2020.
- ^ “Information for Healthcare Professionals on Pfizer/BioNTech COVID-19 vaccine”. Government of the United Kingdom. Retrieved 29 January 2021.
- ^ “Recommendation for an Emergency Use Listing of Tozinameran (Covid-19 Mrna Vaccine (Nucleoside Modified)) Submitted by Biontech Manufacturing Gmbh” (PDF). World Health Organization. 26 January 2021.
- ^ “Australian Product Information – Comirnaty (BNT162b2 [mRNA]) COVID-19 Vaccine”(PDF). Therapeutic Goods Administration. Australian Government.
- ^ “Pfizer and BioNTech Submit COVID-19 Vaccine Stability Data at Standard Freezer Temperature to the U.S. FDA”. Pfizer (Press release). 19 February 2021. Retrieved 19 February 2021.
- ^ Gates B (30 April 2020). “The vaccine race explained: What you need to know about the COVID-19 vaccine”. The Gates Notes. Archived from the original on 14 May 2020. Retrieved 2 May 2020.
- ^ “World Health Organization timeline – COVID-19”. World Health Organization. 27 April 2020. Archived from the original on 29 April 2020. Retrieved 2 May 2020.
- ^ Polack FP, Thomas SJ, Kitchin N, Absalon J, Gurtman A, Lockhart S, et al. (December 2020). “Safety and Efficacy of the BNT162b2 mRNA Covid-19 Vaccine”. The New England Journal of Medicine. 383 (27): 2603–2615. doi:10.1056/NEJMoa2034577. PMC 7745181. PMID 33301246.
development of BNT162b2 was initiated on January 10, 2020, when the SARS-CoV-2 genetic sequence was released by the Chinese Center for Disease Control and Prevention and disseminated globally by the GISAID (Global Initiative on Sharing All Influenza Data) initiative
- ^ Bohn MK, Mancini N, Loh TP, Wang CB, Grimmler M, Gramegna M, et al. (October 2020). “IFCC Interim Guidelines on Molecular Testing of SARS-CoV-2 Infection”. Clinical Chemistry and Laboratory Medicine. 58 (12): 1993–2000. doi:10.1515/cclm-2020-1412. PMID 33027042.
- ^ “CEPI’s collaborative task force to assess COVID-19 vaccines on emerging viral strains”. BioSpectrum – Asia Edition. 23 November 2020.
the first SARS-CoV-2 viral genomes were shared via GISAID on 10 January 2020
- ^ Thanh Le T, Andreadakis Z, Kumar A, Gómez Román R, Tollefsen S, Saville M, Mayhew S (May 2020). “The COVID-19 vaccine development landscape”. Nature Reviews. Drug Discovery. 19 (5): 305–306. doi:10.1038/d41573-020-00073-5. PMID 32273591.
- ^ Fauci AS, Lane HC, Redfield RR (March 2020). “Covid-19 – Navigating the Uncharted”. The New England Journal of Medicine. 382 (13): 1268–1269. doi:10.1056/nejme2002387. PMC 7121221. PMID 32109011.
- ^ Papadopoulos C (14 December 2020). “Chronologie – So entstand der Corona-Impfstoff von Biontech” [Chronology – That’s how the Covid-vaccine of Biontech was being developed] (in German). Südwestrundfunk. Retrieved 20 December 2020.
- ^ 《Fosun Pharma and BioNTech form COVID‑19 vaccine strategic alliance in China》(Fosun Phrama News Content , 15 March 2020) Archived 15 August 2020 at the Wayback Machine
- ^ “Germany: Investment Plan for Europe – EIB to provide BioNTech with up to €100 million in debt financing for COVID-19 vaccine development and manufacturing”. European Investment Bank. 11 June 2020. Archived from the original on 9 November 2020. Retrieved 10 November 2020.
- ^ “BioNTech gets $445 million in German funding for vaccine”. Bloomberg L.P. 15 September 2020. Archived from the original on 9 November 2020. Retrieved 10 November 2020.
- ^ “Pfizer CEO says he would’ve released vaccine data before election if possible”. Axios. 9 November 2020. Archived from the original on 10 November 2020. Retrieved 11 November 2020.
- ^ Jump up to:a b Mulligan MJ, Lyke KE, Kitchin N, Absalon J, Gurtman A, Lockhart S, et al. (October 2020). “Phase I/II study of COVID-19 RNA vaccine BNT162b1 in adults”. Nature. 586(7830): 589–593. Bibcode:2020Natur.586..589M. doi:10.1038/s41586-020-2639-4. PMID 32785213. S2CID 221126922.
- ^ Hannah B (7 October 2020). “EMA begins rolling review of BNT162b2 COVID-19 vaccine”. European Pharmaceutical Review. Archived from the original on 11 November 2020. Retrieved 11 November 2020.
- ^ Jump up to:a b “EMA Assessment Report” (PDF). Europa (web portal). 21 December 2020. Retrieved 29 December 2020.
- ^ “Clarification of Comirnaty dosage interval”. European Medicines Agency (EMA). 28 January 2021. Retrieved 28 January 2021.
- ^ “Study to Evaluate the Safety, Tolerability, and Immunogenicity of SARS CoV-2 RNA Vaccine Candidate (BNT162b2) Against COVID-19 in Healthy Pregnant Women 18 Years of Age and Older”. ClinicalTrials.gov. Retrieved 21 February 2021.
- ^ “Pfizer and BioNTech Commence Global Clinical Trial to Evaluate COVID-19 Vaccine in Pregnant Women”. pfizer.com (Press release). 18 February 2021. Retrieved 21 February2021.
- ^ Jump up to:a b Gaebler C, Nussenzweig MC (October 2020). “All eyes on a hurdle race for a SARS-CoV-2 vaccine”. Nature. 586 (7830): 501–2. Bibcode:2020Natur.586..501G. doi:10.1038/d41586-020-02926-w. PMID 33077943. S2CID 224808629.
- ^ Jump up to:a b “China’s Fosun to end BioNTech’s COVID-19 vaccine trial, seek approval for another”. Reuters. 3 November 2020. Archived from the original on 12 December 2020. Retrieved 21 November 2020.
- ^ Jump up to:a b c World Health Organization. “Messenger RNA encoding the full-length SARS-CoV-2 spike glycoprotein” (DOC). WHO MedNet. Retrieved 16 December 2020.
- ^ Pallesen J, Wang N, Corbett KS, Wrapp D, Kirchdoerfer RN, Turner HL, et al. (August 2017). “Immunogenicity and structures of a rationally designed prefusion MERS-CoV spike antigen”. Proceedings of the National Academy of Sciences of the United States of America. 114 (35): E7348–E7357. doi:10.1073/pnas.1707304114. PMC 5584442. PMID 28807998.
- ^ Orlandini von Niessen AG, Poleganov MA, Rechner C, Plaschke A, Kranz LM, Fesser S, et al. (April 2019). “Improving mRNA-Based Therapeutic Gene Delivery by Expression-Augmenting 3′ UTRs Identified by Cellular Library Screening”. Molecular Therapy. 27 (4): 824–836. doi:10.1016/j.ymthe.2018.12.011. PMC 6453560. PMID 30638957.
- ^ Jump up to:a b “Pfizer-BioNTech COVID-19 vaccine: Health Canada recommendations for people with serious allergies”. Health Canada. 12 December 2020.
- ^ Jump up to:a b c d Comirnaty: Product Information (PDF) (Report). European Medicines Agency(EMA). Retrieved 23 December 2020.
- ^ Public Assessment Report Authorisation for Temporary Supply COVID-19 mRNA Vaccine BNT162b2 (BNT162b2 RNA) concentrate for solution for injection (PDF). Regulation 174(Report). Medicines and Healthcare products Regulatory Agency (MHRA). 15 December 2020.
- ^ “UK medicines regulator gives approval for first UK COVID-19 vaccine”. Medicines and Healthcare products Regulatory Agency (MHRA). 2 December 2020. Archived from the original on 2 December 2020. Retrieved 2 December 2020.
- ^ Neergaard L, Kirka D (2 December 2020). “Britain OKs Pfizer vaccine and will begin shots within days”. Associated Press. Archived from the original on 6 December 2020. Retrieved 6 December 2020.
- ^ Mueller B (2 December 2020). “U.K. Approves Pfizer Coronavirus Vaccine, a First in the West”. The New York Times. Retrieved 2 December 2020.
- ^ Roberts M (2 December 2020). “Covid Pfizer vaccine approved for use next week in UK”. BBC News. Archived from the original on 2 December 2020. Retrieved 2 December 2020.
- ^ Henley J, Connolly, Jones S (3 December 2020). “European and US experts question UK’s fast-track of Covid vaccine”. The Guardian. Archived from the original on 9 December 2020. Retrieved 9 December 2020.
- ^ “First patient receives Pfizer Covid-19 vaccine”. BBC. 8 December 2020. Archivedfrom the original on 8 December 2020. Retrieved 8 December 2020.
- ^ “Vaccine vials and a virtual hug: a history of coronavirus in 15 objects”. The Guardian. 21 February 2021. Retrieved 22 February 2021.
- ^ “COVID-19 Vaccination Statistics –Week ending Sunday 20th December 2020” (PDF). NHS. 24 December 2020.
- ^ “Coronavirus en la Argentina: La ANMAT aprobo el uso de emergencia de la vacuna Pfizer”. La Nación (in Spanish). Retrieved 25 December 2020.
- ^ “TGA provisionally approves Pfizer COVID-19 vaccine”. Therapeutic Goods Administration (Press release). 25 January 2021. Retrieved 26 January 2021.
- ^ “Bahrain becomes second country to approve Pfizer COVID-19 vaccine”. Al Jazeera. Retrieved 5 December 2020.
- ^ “Drug and vaccine authorizations for COVID-19: List of applications received”. Health Canada. 9 December 2020. Retrieved 9 December 2020.
- ^ Jump up to:a b “Chile y Ecuador se adelantan en Sudamérica y autorizan la vacuna de Pfizer”. El Pais. Retrieved 17 December 2020.
- ^ “First Pfizer COVID-19 vaccines set to reach Costa Rica on Wednesday – president”. Reuters. 23 December 2020. Retrieved 24 December 2020.
- ^ “SFH authorises COVID-19 vaccine by Fosun Pharma/BioNTech for emergency use in Hong Kong”. The Government of Hong Kong (Press release). 25 January 2021. Retrieved 26 January 2021.
- ^ “Iraq grants emergency approval for Pfizer COVID-19 vaccine”. MSN. Retrieved 27 December 2020.
- ^ “Israeli Health Minister ‘pleased’ as FDA approves Pfizer COVID-19 vaccine”. The Jerusalem Post. Retrieved 28 December 2020.
- ^ “Jordan approves Pfizer-BioNTech Covid vaccine”. France 24. 15 December 2020. Retrieved 15 December 2020.
- ^ “Kuwait authorizes emergency use of Pfizer-BioNTech COVID-19 vaccine”. Arab News. 13 December 2020. Retrieved 15 December 2020.
- ^ “Mexico Approves Pfizer Vaccine for Emergency Use as Covid Surges”. Bloomberg. 12 December 2020. Retrieved 12 December 2020.
- ^ “Oman issues licence to import Pfizer BioNTech Covid vaccine – TV”. Reuters. 15 December 2020. Retrieved 16 December 2020.
- ^ “Panama approves Pfizer’s COVID-19 vaccine – health ministry”. Yahoo! Finance. Retrieved 16 December 2020.
- ^ “PH authorizes Pfizer’s COVID-19 vaccine for emergency use”. CNN Philippines. 14 January 2021.
- ^ “Qatar, Oman to receive Pfizer-BioNTech COVID-19 vaccine this week”. Reuters. Retrieved 24 December 2020.
- ^ “Saudi Arabia to Launch Its Coronavirus Vaccination Program” (in Spanish). Boomberg. Retrieved 17 December 2020.
- ^ Abdullah Z (14 December 2020). “Pfizer-BioNTech COVID-19 vaccine approved by Singapore, first shipment expected by end-December”. CNA. Retrieved 16 January 2021.
- ^ “Singapore approves use of Pfizer’s COVID-19 vaccine”. AP News. 14 December 2020. Retrieved 15 December 2020.
- ^ “Dubai approves the Pfizer-BioNTech vaccine which will be free of charge”. Emirates Woman. 23 December 2020. Retrieved 28 December 2020.
- ^ “WHO issues its first emergency use validation for a COVID-19 vaccine and emphasizes need for equitable global access”. World Health Organization (WHO) (Press release). 31 December 2020. Retrieved 6 January 2021.
- ^ Jump up to:a b “Emergency Use Authorization for vaccines explained”. U.S. Food and Drug Administration (FDA). 20 November 2020. Archived from the original on 20 November 2020. Retrieved 20 November 2020.
This article incorporates text from this source, which is in the public domain.
- ^ “Pfizer-BioNTech COVID-19 Vaccine EUA Letter of Authorization” (PDF). U.S. Food and Drug Administration (FDA). 11 December 2020.
This article incorporates text from this source, which is in the public domain.
- ^ Jump up to:a b c d “Pfizer-BioNTech COVID-19 Vaccine EUA Fact Sheet for Healthcare Providers”(PDF). Pfizer. 11 December 2020.
- ^ Sun LH, Stanley-Becker I. “CDC greenlights advisory group’s decision to recommend Pfizer vaccine for use”. The Washington Post. Retrieved 14 December 2020.
- ^ Oliver SE, Gargano JW, Marin M, Wallace M, Curran KG, Chamberland M, et al. (December 2020). “The Advisory Committee on Immunization Practices’ Interim Recommendation for Use of Pfizer-BioNTech COVID-19 Vaccine — United States, December 2020” (PDF). MMWR. Morbidity and Mortality Weekly Report. 69 (50): 1922–24. doi:10.15585/mmwr.mm6950e2. PMC 7745957. PMID 33332292.
- ^ “COVID-19: Switzerland can start vaccinating vulnerable groups already in December”(Press release). Federal Office of Public Health. 19 December 2020. Retrieved 19 December 2020.
- ^ Erni S (23 December 2020). “90-jährige Luzernerin als erste Person in der Schweiz gegen Corona geimpft”. Neue Luzerner Zeitung. Retrieved 23 December 2020.
- ^ Pralong J (23 December 2020). “La piqûre de l’espoir pratiquée à Lucerne”. Heidi.news. Retrieved 23 December 2020.
- ^ Jump up to:a b “EMA recommends first COVID-19 vaccine for authorisation in the EU”. European Medicines Agency (EMA) (Press release). 21 December 2020. Retrieved 21 December2020.
- ^ “Comirnaty”. Union Register of medicinal products. Retrieved 8 January 2021.
- ^ “Statement by President von der Leyen on the marketing authorisation of the BioNTech-Pfizer vaccine against COVID-19”. European Commission. Retrieved 21 December 2020.
- ^ Cancian, Natália (23 February 2021). “Anvisa aprova registro da vacina da Pfizer contra Covid”. Folha de S. Paulo (in Portuguese). Retrieved 23 February 2021.
- ^ McKenna M (17 December 2020). “Vaccines Are Here. We Have to Talk About Side Effects”. Wired. Retrieved 23 December 2020.
- ^ Jump up to:a b CDC COVID-19 Response Team, Food and Drug Administration (January 2021). “Allergic Reactions Including Anaphylaxis After Receipt of the First Dose of Pfizer-BioNTech COVID-19 Vaccine — United States, December 14–23, 2020” (PDF). MMWR. Morbidity and Mortality Weekly Report. 70 (2): 46–51. doi:10.15585/mmwr.mm7002e1. PMC 7808711. PMID 33444297.
- ^ Jump up to:a b c “COVID-19 vaccine safety update: COMIRNATY” (PDF). European Medicines Agency. 28 January 2021.
- ^ Bostock N (9 December 2020). “MHRA warning after allergic reactions in NHS staff given COVID-19 vaccine”. GP. Archived from the original on 9 December 2020. Retrieved 9 December 2020.
- ^ Booth W, Cunningham E (9 December 2020). “Britain warns against Pfizer vaccine for people with history of ‘significant’ allergic reactions”. The Washington Post. Archivedfrom the original on 9 December 2020. Retrieved 9 December 2020.
- ^ Cabanillas B, Akdis C, Novak N (December 2020). “Allergic reactions to the first COVID-19 vaccine: a potential role of Polyethylene glycol?”. Allergy. doi:10.1111/all.14711. PMID 33320974. S2CID 229284320.
- ^ “First COVID-19 vaccine safety update published”. European Medicines Agency (EMA)(Press release). 28 January 2021. Retrieved 29 January 2021.
- ^ Gray B (23 November 2020). “Pfizer’s Chesterfield workforce playing a key role in coronavirus vaccine development”. St. Louis Post-Dispatch.
- ^ Jump up to:a b c d Johnson CY (17 November 2020). “A vial, a vaccine and hopes for slowing a pandemic — how a shot comes to be”. The Washington Post. Retrieved 21 December2020.
- ^ Hughes M (20 December 2020). “Andover’s piece of the vaccine: Pfizer”. The Eagle-Tribune.
- ^ Shamus KJ (13 December 2020). “Historic journey: Pfizer prepares to deliver 6.4 million doses of COVID-19 vaccines”. Detroit Free Press.
- ^ Mullin R (25 November 2020). “Pfizer, Moderna ready vaccine manufacturing networks”. Chemical & Engineering News. Washington, D.C.: American Chemical Society. Retrieved 21 December 2020.
- ^ Jump up to:a b c Weise, Elizabeth (7 February 2021). “Pfizer expects to cut COVID-19 vaccine production time by close to 50% as production ramps up, efficiencies increase”. USA Today.
- ^ “BioNTech to Acquire GMP Manufacturing Site to Expand COVID-19 Vaccine Production Capacity in First Half 2021 | BioNTech”. investors.biontech.de. Retrieved 5 February2021.
- ^ “Statement on Manufacturing | BioNTech”. investors.biontech.de. Retrieved 5 February2021.
- ^ Erman M, Ankur B (22 July 2020). “U.S. to pay Pfizer, BioNTech $1.95 bln for millions of COVID-19 vaccine doses”. Reuters. Archived from the original on 22 July 2020. Retrieved 22 July 2020.
- ^ “U.S. Government Engages Pfizer to Produce Millions of Doses of COVID-19 Vaccine”. US Department of Health and Human Services. 22 July 2020. Archived from the original on 22 July 2020. Retrieved 23 July 2020.
- ^ Nazaryan A (9 November 2020). “So is Pfizer part of Operation Warp Speed or not? Yes and no”. Yahoo!. Archived from the original on 10 November 2020. Retrieved 9 November 2020.
- ^ Pleitgen F (11 November 2020). “EU agrees to buy 300 million doses of the Pfizer/BioNTech Covid-19 vaccine”. CNN. Archived from the original on 24 November 2020. Retrieved 26 November 2020.
- ^ “Japan and Pfizer reach COVID-19 vaccine deal to treat 60 million people”. The Japan Times. 1 August 2020. Archived from the original on 10 November 2020. Retrieved 21 November 2020.
- ^ Tasker JP (9 November 2020). “Trudeau says promising new Pfizer vaccine could be ‘light at the end of the tunnel'”. CBC News. Archived from the original on 9 November 2020. Retrieved 9 November 2020.
- ^ “Pfizer and BioNTech to Supply Singapore with their BNT162b2 mRNA-based Vaccine Candidate to Combat COVID-19”. pfizer.com.sg. Pfizer Singapore. 14 December 2020. Retrieved 1 February 2021.
- ^ de Salud S. “233. Firma secretario de Salud convenio con Pfizer para fabricación y suministro de vacuna COVID-19”. gob.mx (in Spanish). Retrieved 17 December 2020.
- ^ Ng E (27 August 2020). “Fosun Pharma to supply Covid-19 vaccine to Hong Kong, Macau once approved”. South China Morning Post. Archived from the original on 20 November 2020. Retrieved 21 November 2020.
- ^ Ting V, Lau C, Wong O (11 December 2020). “Hong Kong buys 15 million Covid-19 vaccine doses from Sinovac, Pfizer”. South China Morning Post. Retrieved 18 December2020.
- ^ “BioNTech and Fosun Pharma to Supply China with mRNA-based COVID-19 Vaccine”(Press release). BioNTech. 16 December 2020. Retrieved 16 December 2020.
- ^ “Pfizer-BioNTech COVID-19 Vaccine Frequently Asked Questions”. U.S. Food and Drug Administration. 11 December 2020. Retrieved 29 December 2020.
This article incorporates text from this source, which is in the public domain.
- ^ Jump up to:a b “Extra dose from vials of Comirnaty COVID-19 vaccine”. European Medicines Agency (EMA). 8 January 2021. Retrieved 8 January 2021.
- ^ “AIFA, possibile ottenere almeno 6 dosi da ogni flaconcino del vaccino BioNTech/Pfizer”. aifa.gov.it (in Italian). Retrieved 29 December 2020.
- ^ “Global information about Comirnaty”. Comirnaty IE. 8 January 2021. Retrieved 16 January 2021.
- ^ “Comirnaty Package Insert” (PDF). BioNTech Manufacturing GmbH.
- ^ Rowland C (22 January 2021). “Biden wants to squeeze an extra shot of vaccine out of every Pfizer vial. It won’t be easy”. The Washington Post. Retrieved 29 January 2021.
- ^ Kollewe J. “Pfizer and BioNTech’s vaccine poses global logistics challenge”. The Guardian. Archived from the original on 10 November 2020. Retrieved 10 November2020.
- ^ Newey S (8 September 2020). “Daunting task of distribution exposed as it emerges some vaccines must be ‘deep frozen’ at −70C”. The Telegraph. Archived from the original on 9 November 2020. Retrieved 10 November 2020.
- ^ “How China’s COVID-19 could fill the gaps left by Pfizer, Moderna, AstraZeneca”. Fortune. 5 December 2020. Archived from the original on 12 December 2020. Retrieved 5 December 2020.
- ^ “Pfizer’s Vaccine Is Out of the Question as Indonesia Lacks Refrigerators: State Pharma Boss”. Jakarta Globe. 22 November 2020. Archived from the original on 7 December 2020. Retrieved 5 December 2020.
- ^ “Pfizer Has Offered South Africa Discounted Covid-19 Vaccines”. Bloomberg. 4 January 2021. Retrieved 5 January 2021.
- ^ Polack FP, Thomas SJ, Kitchin N, Absalon J, Gurtman A, Lockhart S, et al. (December 2020). “Safety and Efficacy of the BNT162b2 mRNA Covid-19 Vaccine”. N Engl J Med. 383 (27): 2603–2615. doi:10.1056/NEJMoa2034577. PMC 7745181. PMID 33301246.
- ^ World Health Organization (2020). “International Nonproprietary Names for Pharmaceutical Substances (INN). Proposed INN: List 124 – COVID-19 (special edition)”(PDF). WHO Drug Information. 34 (3): 666. Archived (PDF) from the original on 27 November 2020. Retrieved 23 November 2020.
- ^ “Pfizer and BioNTech Receive Authorization in the European Union for COVID-19 Vaccine” (Press release). BioNTech. 21 December 2020. Retrieved 26 December 2020 – via GlobeNewswire.
- ^ Bulik BS (23 December 2020). “The inside story behind Pfizer and BioNTech’s new vaccine brand name, Comirnaty”. FiercePharma. Retrieved 25 December 2020.
- ^ “Comirnaty COVID-19 mRNA Vaccine”. Comirnaty Global. Retrieved 31 December2020.
External links
“Tozinameran”. Drug Information Portal. U.S. National Library of Medicine.
- Global Information About Pfizer–BioNTech COVID‑19 Vaccine (also known as BNT162b2) Pfizer
- Comirnaty assessment report European Medicines Agency Committee for Medicinal Products for Human Use
- A Phase 1/2/3 Study to Evaluate the Safety, Tolerability, Immunogenicity, and Efficacy of RNA Vaccine Candidates Against COVID‑19 in Healthy Individuals Pfizer clinical protocol
- Pfizer Vaccince News, updates and tracking of Israel’s vaccinaion campaign
- “How the Pfizer-BioNTech Covid-19 Vaccine Works”. The New York Times.
A vial of the Pfizer–BioNTech COVID‑19 vaccine | |
Vaccine description | |
---|---|
Target disease | COVID‑19 |
Type | mRNA |
Clinical data | |
Trade names | Comirnaty[1][2] |
Other names | BNT162b2, COVID-19 mRNA vaccine (nucleoside-modified) |
License data | EU EMA: by INNUS DailyMed: Pfizer-BioNTech_COVID-19_Vaccine |
Pregnancy category | AU: B1[3] |
Routes of administration | Intramuscular |
ATC code | None |
Legal status | |
Legal status | AU: S4 (Prescription only) [4][5]CA: Authorized by interim order [6][7]UK: Conditional and temporary authorization to supply [8][9]US: Unapproved (Emergency Use Authorization)[10][11][12]EU: Conditional marketing authorization granted [2]CH: Rx-only[further explanation needed][1] |
Identifiers | |
CAS Number | 2417899-77-3 |
PubChem SID | 434370509 |
DrugBank | DB15696 |
UNII | 5085ZFP6SJ |
KEGG | D11971 |
Part of a series on the |
COVID-19 pandemic |
---|
SARS-CoV-2 (virus)COVID-19 (disease) |
showTimeline |
showLocations |
showInternational response |
showMedical response |
showImpact |
COVID-19 Portal |
/////////
#Tozinameran, #APPROVALS 2021, #JAPAN 2021, Comirnaty, #Coronavirus disease, #COVID-19, #BNT162b2 , #BNT162b2, #SARS-CoV-2 Vaccine, #RNA ingredient BNT-162B2, #corona
The Pfizer-BioNTech COVID-19 vaccine (Tozinameran, INN), also known as BNT162b2, is one of four advanced mRNA-based vaccines developed through “Project Lightspeed,” a joint program between Pfizer and BioNTech.2,3 Tozinameran is a nucleoside modified mRNA (modRNA) vaccine encoding an optimized full-length version of the severe acute respiratory syndrome coronavirus 2 (SARS-CoV-2) spike (S) protein. It is designed to induce immunity against SARS-CoV-2, the virus responsible for causing COVID-19.2 The modRNA is formulated in lipid nanoparticles for administration via intramuscular injection in two doses, three weeks apart.1,3
Tozinameran is undergoing evaluation in clinical trials in both the USA (NCT04368728) and Germany (NCT04380701).4,5 Tozinameran received fast track designation by the U.S. FDA on July 13, 2020.6 On December 11, 2020, the FDA issued an Emergency Use Authorization (EUA) based on 95% efficacy in clinical trials and a similar safety profile to other viral vaccines over a span of approximately 2 months.1 Tozinameran was granted an EUA in the UK on December 2, 2020,8 and in Canada on December 9, 20207 for active immunization against SARS-CoV-2.12
Currently, sufficient data are not available to determine the longevity of protection against COVID-19, nor direct evidence that the vaccine prevents the transmission of the SARS-CoV-2 virus from one individual to another.9 Fact sheets for caregivers, recipients, and healthcare providers are now available.10,11
Tozinameran has not yet been fully approved by any country. In both the UK and Canada, Tozinameran is indicated under an interim authorization for active immunization to prevent COVID-19 caused by SARS-CoV-2 in individuals aged 16 years and older.7,8
On December 11, 2020, the U.S. Food and Drug Administration granted emergency use authorization (EUA) for Tozinameran to prevent COVID-19 caused by severe acute respiratory syndrome coronavirus 2 (SARS-CoV-2) in patients aged 16 years and above.9 Safety and immune response information for adolescents 12-15 years of age will follow, and studies to further explore the administration of Tozinameran in pregnant women, children under 12 years of age, and those in special risk groups will be evaluated in the future.1
This vaccine should only be administered where appropriate medical treatment for immediate allergic reactions are immediately available in the case of an acute anaphylactic reaction after vaccine administration.12 Tozinameran administration should be postponed in any individual suffering from an acute febrile illness. Its use should be carefully considered in immunocompromised individuals and individuals with a bleeding disorder or on anticoagulant therapy. Appropriate medical treatment should be readily available in case of an anaphylactic reaction following vaccine administration.7,8
Tozinameran contains nucleoside modified mRNA (modRNA) encapsulated in lipid nanoparticles that deliver the modRNA into host cells. The lipid nanoparticle formulation facilitates the delivery of the RNA into human cells.12 Once inside these cells, the modRNA is translated by host machinery to produce the SARS-CoV-2 spike (S) protein antigen, which is subsequently recognized by the host immune system. Tozinameran has been shown to elicit both neutralizing antibody and cellular immune responses to the S protein, which helps protect against subsequent SARS-CoV-2 infection.7,8
Tozinameran is a nucleoside modified mRNA (modRNA) vaccine encoding an optimized full-length version of the SARS-CoV-2 spike (S) protein, translated and expressed in cells in vaccinated individuals to produce the S protein antigen against which an immune response is mounted. As with all vaccines, protection cannot be guaranteed in all recipients, and full protection may not occur until at least seven days following the second dose.7,8
In U.S. clinical trials, the vaccine was 95% effective in preventing COVID-19; eight COVID-19 cases occurred in the vaccine group and 162 cases occurred in the placebo group. Of the total 170 COVID-19 cases, one case in the vaccine group and three cases in the placebo group were considered to be severe infections.1,9
- Polack FP, Thomas SJ, Kitchin N, Absalon J, Gurtman A, Lockhart S, Perez JL, Perez Marc G, Moreira ED, Zerbini C, Bailey R, Swanson KA, Roychoudhury S, Koury K, Li P, Kalina WV, Cooper D, Frenck RW Jr, Hammitt LL, Tureci O, Nell H, Schaefer A, Unal S, Tresnan DB, Mather S, Dormitzer PR, Sahin U, Jansen KU, Gruber WC: Safety and Efficacy of the BNT162b2 mRNA Covid-19 Vaccine. N Engl J Med. 2020 Dec 10. doi: 10.1056/NEJMoa2034577. [PubMed:33301246]
- Gen Eng News: BNT162 vaccine candidates [Link]
- BioNTech BNT162 Update [Link]
- Clinical Trial NCT04368728 [Link]
- Clinical Trial NCT04380701 [Link]
- FDA fast track designation: BNT162b1 and BNT162b2 [Link]
- Health Canada Interim Product Monograph: BNT162b2 SARS-CoV-2 Vaccine [Link]
- MHRA Interim Product Monograph: BNT162b2 SARS-CoV-2 Vaccine [Link]
- FDA News Release: FDA Takes Key Action in Fight Against COVID-19 By Issuing Emergency Use Authorization for First COVID-19 Vaccine [Link]
- Pfizer: Fact Sheet for Healthcare Providers Administering Vaccine, Pfizer-BioNtech COVID-19 vaccine [Link]
- Pfizer: Fact Sheet for Recipients and Caregivers, Pfizer BioNTech COVID-19 vaccine [Link]
- FDA Emergency Use Authorization: Full EUA Prescribing information, Pfizer-BioNTech COVID-19 vaccine [Link]
-
PHASESTATUSPURPOSECONDITIONSCOUNT2Active Not RecruitingPreventionCoronavirus Disease 2019 (COVID‑19)12, 3Active Not RecruitingPreventionCoronavirus Disease 2019 (COVID‑19)11, 2Active Not RecruitingPreventionCoronavirus Disease 2019 (COVID‑19)11, 2RecruitingTreatmentCoronavirus Disease 2019 (COVID‑19) / Protection Against COVID-19 and Infections With SARS CoV 2 / Respiratory Tract Infections (RTI) / RNA Virus Infections / Vaccine Adverse Reaction / Viral Infections / Virus Diseases1
Desidustat
Ranjit Desai
DESIDUSTAT
2-(1-(cyclopropylmethoxy)-4-hydroxy-2-oxo-1,2-dihydroquinoline-3-carboxamido)acetic acid
desidustat
Glycine, N-((1-(cyclopropylmethoxy)-1,2-dihydro-4-hydroxy-2-oxo-3-quinolinyl)carbonyl)-
N-(1-(Cyclopropylmethoxy)-4-hydroxy-2-oxo-1,2-dihydroquinoline-3-carbonyl)glycine
ZYAN1 compound
(1-(cyclopropylmethoxy)-4-hydroxy-2-oxo-1,2-dihydroquinoline-3-carbonyl) glycine in 98% yield, as a solid. MS (ESI-MS): m/z 333.05 (M+H) +. 1H NMR (DMSO-d 6): 0.44-0.38 (m, 2H), 0.62-0.53 (m, 2H), 1.34-1.24 (m, 1H), 4.06-4.04 (d, 2H), 4.14-4.13 (d, 2H), 7.43-7.39 (t, 1H), 7.72-7.70 (d, 1H), 7.89-7.85 (m, 1H), 8.11-8.09 (dd, 1H), 10.27-10.24 (t, 1H), 12.97 (bs, 1H), 16.99 (s, 1H). HPLC Purity: 99.85%
Oxemia (Desidustat) has received approval from the Drug Controller General of India. This was an incredible team effort by Zydans across the organization and I am so proud of what we have accomplished. Oxemia is a breakthrough treatment for Anemia associated with Chronic Kidney Disease in Patients either on Dialysis or Not on Dialysis, and will help improve quality of life for CKD patients. Team #zydus , on to our next effort!
Desidustat (INN, also known as ZYAN1) is a drug for the treatment of anemia of chronic kidney disease. This drug with the brand name Oxemia is discovered and developed by Zydus Life Sciences.[1] The subject expert committee of CDSCO has recommended the grant of permission for manufacturing and marketing of Desidustat 25 mg and 50 mg tablets in India,based on some conditions related to package insert, phase 4 protocols, prescription details, and GCP.[2] Clinical trials on desidustat have been done in India and Australia.[3] In a Phase 2, randomized, double-blind, 6-week, placebo-controlled, dose-ranging, safety and efficacy study, a mean hemoglobin increase of 1.57, 2.22, and 2.92 g/dL in desidustat 100, 150, and 200 mg arms, respectively, was observed.[4] The Phase 3 clinical trials were conducted at additional lower doses as of 2019.[5] Desidustat is developed for the treatment of anemia as an oral tablet, where currently injections of erythropoietin and its analogues are drugs of choice. Desidustat is a HIF prolyl-hydroxylase inhibitor. In preclinical studies, effects of desidustat was assessed in normal and nephrectomized rats, and in chemotherapy-induced anemia. Desidustat demonstrated hematinic potential by combined effects on endogenous erythropoietin release and efficient iron utilization.[6][7] Desidustat can also be useful in treatment of anemia of inflammation since it causes efficient erythropoiesis and hepcidin downregulation.[8] In January 2020, Zydus entered into licensing agreement with China Medical System (CMS) Holdings for development and commercialization of desidustat in Greater China. Under the license agreement, CMS will pay Zydus an initial upfront payment, regulatory milestones, sales milestones and royalties on net sales of the product. CMS will be responsible for development, registration and commercialization of desidustat in Greater China.[9] It has been observed that desidustat protects against acute and chronic kidney injury by reducing inflammatory cytokines like IL-6 and oxidative stress [10] A clinical trial to evaluate the efficacy and safety of desidustat tablet for the management of Covid-19 patients is ongoing in Mexico, wherein desidustat has shown to prevent acute respiratory distress syndrome (ARDS) by inhibiting IL-6.[11] Zydus has also received approval from the US FDA to initiate clinical trials of desidustat in chemotherapy Induced anemia (CIA).[12]. Desidustat has met the primary endpoints in the phase 3 clinical trials and Zydus had filed the New Drug Application (NDA) to DCGI in November, 2021.[13]\
CLIP
Zydus receives DCGI approval for new drug Oxemia; what you need to know
The new drug is an oral, small molecule hypoxia-inducible factor-prolyl hydroxylase (HIF-PH) inhibitor, Zydus said in a statement.
Gujarat-based pharma company Zydus Lifesciences on Monday received the Drugs Controller General of India (DCGI) approval for its new drug application for a first-of-its-kind oral treatment for anemia associated with Chronic Kidney Disease (CKD) – Oxemia (Desidustat).
The new drug is an oral, small molecule hypoxia-inducible factor-prolyl hydroxylase (HIF-PH) inhibitor, the drug firm said in a statement.
Desidustat showed good safety profile, improved iron mobilization and LDL-C reduction in CKD patients in DREAM-D and DREAM-ND Phase III clinical trials, conducted in approximately 1,200 subjects. Desidustat provides CKD patients with an oral convenient therapeutic option for the treatment of anemia. The pharma major did not, however, declare the cost per dose if the drug is available in the market.
“After more than a decade of research and development into the science of HIF-PH inhibitors, results have demonstrated that Oxemia addresses this unmet need and additionally reduces hepcidin, inflammation and enables better iron mobilization. This advancement offers ease of convenience for the patient and will also reduce the disease burden by providing treatment at an affordable cost, thereby improving the quality of life for patients suffering from Chronic Kidney Disease,” Chairman of Zydus Lifesciences Pankaj Patel said.
Chronic Kidney Disease (CKD) is a progressive medical condition characterised by a gradual loss of kidney function and is accompanied by comorbidities like anemia, cardiovascular diseases (hypertension, heart failure and stroke), diabetes mellitus, eventually leading to kidney failure.
PATENT
Scheme 3:
|
Step 1′a Process for Preparation of ethyl 2-iodobenzoate (XI-a)
Step-2 Process for the Preparation of ethyl 2-((tert-butoxycarbonyl)(cyclopropylmethoxy)aminolbenzoate (XII-a)
Step 3 Process for the Preparation of ethyl 2-((cyclopropylmethoxy)amino)benzoate (XIII-a)
Step 4 Process for the Preparation of ethyl 24N-(cyclopropylinethoxy)-3-ethoxy-3-oxopropanamido)benzoate (XIV-a)
Step 5: Process for the Preparation of ethyl 1-(cyclopropylmethoxy)-4-hydroxy-2-oxo-1,2 dihydroquinolline-3-carboxylate (XY-a)
Purification
Step 6 Process for the Preparation of ethyl (1-(cyclopropylmethoxy)-4-hydroxy-2-oxo-1,2-dihydroquinoline-3-carbonyl)glycinate (XVI-a)
Purification
Step 7: Process for the Preparation of (1-(cyclopropylmethoxy)-4-hydroxy-2-oxo-1,2-dihydroquinoline-3-carbonyl)glycine (I-a)
Polymorphic Data (XRPD):
References[edit]
- ^ “Zydus receives DCGI approval for new drug Oxemia; what you need to know”.
- ^ CDSCO, SEC Committee. “SEC meeting to examine IND proposals, dated 29.12.2021”. CDSCO website Govt of India. CDSCO. Retrieved 19 January 2022.
- ^ Kansagra KA, Parmar D, Jani RH, Srinivas NR, Lickliter J, Patel HV, et al. (January 2018). “Phase I Clinical Study of ZYAN1, A Novel Prolyl-Hydroxylase (PHD) Inhibitor to Evaluate the Safety, Tolerability, and Pharmacokinetics Following Oral Administration in Healthy Volunteers”. Clinical Pharmacokinetics. 57 (1): 87–102. doi:10.1007/s40262-017-0551-3. PMC 5766731. PMID 28508936.
- ^ Parmar DV, Kansagra KA, Patel JC, Joshi SN, Sharma NS, Shelat AD, Patel NB, Nakrani VB, Shaikh FA, Patel HV; on behalf of the ZYAN1 Trial Investigators. Outcomes of Desidustat Treatment in People with Anemia and Chronic Kidney Disease: A Phase 2 Study. Am J Nephrol. 2019 May 21;49(6):470-478. doi: 10.1159/000500232.
- ^ “Zydus Cadila announces phase III clinical trials of Desidustat”. 17 April 2019. Retrieved 20 April 2019 – via The Hindu BusinessLine.
- ^ Jain MR, Joharapurkar AA, Pandya V, Patel V, Joshi J, Kshirsagar S, et al. (February 2016). “Pharmacological Characterization of ZYAN1, a Novel Prolyl Hydroxylase Inhibitor for the Treatment of Anemia”. Drug Research. 66 (2): 107–12. doi:10.1055/s-0035-1554630. PMID 26367279.
- ^ Joharapurkar AA, Pandya VB, Patel VJ, Desai RC, Jain MR (August 2018). “Prolyl Hydroxylase Inhibitors: A Breakthrough in the Therapy of Anemia Associated with Chronic Diseases”. Journal of Medicinal Chemistry. 61 (16): 6964–6982. doi:10.1021/acs.jmedchem.7b01686. PMID 29712435.
- ^ Jain M, Joharapurkar A, Patel V, Kshirsagar S, Sutariya B, Patel M, et al. (January 2019). “Pharmacological inhibition of prolyl hydroxylase protects against inflammation-induced anemia via efficient erythropoiesis and hepcidin downregulation”. European Journal of Pharmacology. 843: 113–120. doi:10.1016/j.ejphar.2018.11.023. PMID 30458168. S2CID 53943666.
- ^ Market, Capital (20 January 2020). “Zydus enters into licensing agreement with China Medical System Holdings”. Business Standard India. Retrieved 20 January 2020 – via Business Standard.
- ^ Joharapurkar, Amit; Patel, Vishal; Kshirsagar, Samadhan; Patel, Maulik; Savsani, Hardikkumar; Jain, Mukul (22 January 2021). “Prolyl hydroxylase inhibitor desidustat protects against acute and chronic kidney injury by reducing inflammatory cytokines and oxidative stress”. Drug Development Research. 82 (6): 852–860. doi:10.1002/ddr.21792. PMID 33480036. S2CID 231680317.
- ^ “Zydus’ trials of Desidustat shows positive results for Covid-19 management”. The Hindu Business Line. The Hindu. Retrieved 25 January 2021.
- ^ “Zydus receives approval from USFDA to initiate clinical trials of Desidustat in cancer patients receiving chemotherapy”. PipelineReview.com. La Merie Publishing. Retrieved 22 January 2021.
- ^ “Stock Share Price | Get Quote | BSE”.
![]() |
|
Clinical data | |
---|---|
Other names | ZYAN1 |
Identifiers | |
CAS Number | |
UNII | |
Chemical and physical data | |
Formula | C16H16N2O6 |
Molar mass | 332.312 g·mol−1 |
3D model (JSmol) | |
Date
CTID | Title | Phase | Status | Date |
---|---|---|---|---|
NCT04215120 | Desidustat in the Treatment of Anemia in CKD on Dialysis Patients | Phase 3 | Recruiting | 2020-01-02 |
NCT04012957 | Desidustat in the Treatment of Anemia in CKD | Phase 3 | Recruiting | 2019-12-24 |
////////// DESIDUSTAT, ZYDUS CADILA, COVID 19, CORONA VIRUS, PHASE 3, ZYAN 1, OXEMIA, APPROVALS 2022, INDIA 2022
AZITHROMYCIN, アジスロマイシン;
AZITHROMYCIN
C38H72N2O12,
748.9845
アジスロマイシン;
CAS: | 83905-01-5 |
PubChem: | 51091811 |
ChEBI: | 2955 |
ChEMBL: | CHEMBL529 |
DrugBank: | DB00207 |
PDB-CCD: | ZIT[PDBj] |
LigandBox: | D07486 |
NIKKAJI: | J134.080H |
Azithromycin is an antibiotic used for the treatment of a number of bacterial infections.[3] This includes middle ear infections, strep throat, pneumonia, traveler’s diarrhea, and certain other intestinal infections.[3] It can also be used for a number of sexually transmitted infections, including chlamydia and gonorrhea infections.[3] Along with other medications, it may also be used for malaria.[3] It can be taken by mouth or intravenously with doses once per day.[3]
Common side effects include nausea, vomiting, diarrhea and upset stomach.[3] An allergic reaction, such as anaphylaxis, QT prolongation, or a type of diarrhea caused by Clostridium difficile is possible.[3] No harm has been found with its use during pregnancy.[3] Its safety during breastfeeding is not confirmed, but it is likely safe.[4] Azithromycin is an azalide, a type of macrolide antibiotic.[3] It works by decreasing the production of protein, thereby stopping bacterial growth.[3]
Azithromycin was discovered 1980 by Pliva, and approved for medical use in 1988.[5][6] It is on the World Health Organization’s List of Essential Medicines, the safest and most effective medicines needed in a health system.[7] The World Health Organization classifies it as critically important for human medicine.[8] It is available as a generic medication[9] and is sold under many trade names worldwide.[2] The wholesale cost in the developing world is about US$0.18 to US$2.98 per dose.[10] In the United States, it is about US$4 for a course of treatment as of 2018.[11] In 2016, it was the 49th most prescribed medication in the United States with more than 15 million prescriptions.[12]
Medical uses
Azithromycin is used to treat many different infections, including:
- Prevention and treatment of acute bacterial exacerbations of chronic obstructive pulmonary disease due to H. influenzae, M. catarrhalis, or S. pneumoniae. The benefits of long-term prophylaxis must be weighed on a patient-by-patient basis against the risk of cardiovascular and other adverse effects.[13]
- Community-acquired pneumonia due to C. pneumoniae, H. influenzae, M. pneumoniae, or S. pneumoniae[14]
- Uncomplicated skin infections due to S. aureus, S. pyogenes, or S. agalactiae
- Urethritis and cervicitis due to C. trachomatis or N. gonorrhoeae. In combination with ceftriaxone, azithromycin is part of the United States Centers for Disease Control-recommended regimen for the treatment of gonorrhea. Azithromycin is active as monotherapy in most cases, but the combination with ceftriaxone is recommended based on the relatively low barrier to resistance development in gonococci and due to frequent co-infection with C. trachomatis and N. gonorrhoeae.[15]
- Trachoma due to C. trachomatis[16]
- Genital ulcer disease (chancroid) in men due to H. ducrey
- Acute bacterial sinusitis due to H. influenzae, M. catarrhalis, or S. pneumoniae. Other agents, such as amoxicillin/clavulanate are generally preferred, however.[17][18]
- Acute otitis media caused by H. influenzae, M. catarrhalis or S. pneumoniae. Azithromycin is not, however, a first-line agent for this condition. Amoxicillin or another beta lactam antibiotic is generally preferred.[19]
- Pharyngitis or tonsillitis caused by S. pyogenes as an alternative to first-line therapy in individuals who cannot use first-line therapy[20]
Bacterial susceptibility
Azithromycin has relatively broad but shallow antibacterial activity. It inhibits some Gram-positive bacteria, some Gram-negative bacteria, and many atypical bacteria.
A strain of gonorrhea reported to be highly resistant to azithromycin was found in the population in 2015. Neisseria gonorrhoeae is normally susceptible to azithromycin,[21] but the drug is not widely used as monotherapy due to a low barrier to resistance development.[15] Extensive use of azithromycin has resulted in growing Streptococcus pneumoniae resistance.[22]
Aerobic and facultative Gram-positive microorganisms
- Staphylococcus aureus (Methicillin-sensitive only)
- Streptococcus agalactiae
- Streptococcus pneumoniae
- Streptococcus pyogenes
Aerobic and facultative Gram-negative microorganisms
- Haemophilus ducreyi
- Haemophilus influenzae
- Moraxella catarrhalis
- Neisseria gonorrhoeae
- Bordetella pertussis
- Legionella pneumophila
Anaerobic microorganisms
- Peptostreptococcus species
- Prevotella bivia
Other microorganisms
- Chlamydophila pneumoniae
- Chlamydia trachomatis
- Mycoplasma genitalium
- Mycoplasma pneumoniae
- Ureaplasma urealyticum
Pregnancy and breastfeeding
No harm has been found with use during pregnancy.[3] However, there are no adequate well-controlled studies in pregnant women.[23]
Safety of the medication during breastfeeding is unclear. It was reported that because only low levels are found in breast milk and the medication has also been used in young children, it is unlikely that breastfed infants would suffer adverse effects.[4] Nevertheless, it is recommended that the drug be used with caution during breastfeeding.[3]
Airway diseases
Azithromycin appears to be effective in the treatment of COPD through its suppression of inflammatory processes.[24] And potentially useful in asthma and sinusitis via this mechanism.[25] Azithromycin is believed to produce its effects through suppressing certain immune responses that may contribute to inflammation of the airways.[26][27]
Adverse effects
Most common adverse effects are diarrhea (5%), nausea (3%), abdominal pain (3%), and vomiting. Fewer than 1% of people stop taking the drug due to side effects. Nervousness, skin reactions, and anaphylaxis have been reported.[28] Clostridium difficile infection has been reported with use of azithromycin.[3] Azithromycin does not affect the efficacy of birth control unlike some other antibiotics such as rifampin. Hearing loss has been reported.[29]
Occasionally, people have developed cholestatic hepatitis or delirium. Accidental intravenous overdose in an infant caused severe heart block, resulting in residual encephalopathy.[30][31]
In 2013 the FDA issued a warning that azithromycin “can cause abnormal changes in the electrical activity of the heart that may lead to a potentially fatal irregular heart rhythm.” The FDA noted in the warning a 2012 study that found the drug may increase the risk of death, especially in those with heart problems, compared with those on other antibiotics such as amoxicillin or no antibiotic. The warning indicated people with preexisting conditions are at particular risk, such as those with QT interval prolongation, low blood levels of potassium or magnesium, a slower than normal heart rate, or those who use certain drugs to treat abnormal heart rhythms.[32][33][34]
Pharmacology
Mechanism of action
Azithromycin prevents bacteria from growing by interfering with their protein synthesis. It binds to the 50S subunit of the bacterial ribosome, thus inhibiting translation of mRNA. Nucleic acid synthesis is not affected.[23]
Pharmacokinetics
Azithromycin is an acid-stable antibiotic, so it can be taken orally with no need of protection from gastric acids. It is readily absorbed, but absorption is greater on an empty stomach. Time to peak concentration (Tmax) in adults is 2.1 to 3.2 hours for oral dosage forms. Due to its high concentration in phagocytes, azithromycin is actively transported to the site of infection. During active phagocytosis, large concentrations are released. The concentration of azithromycin in the tissues can be over 50 times higher than in plasma due to ion trapping and its high lipid solubility.[citation needed] Azithromycin’s half-life allows a large single dose to be administered and yet maintain bacteriostatic levels in the infected tissue for several days.[35]
Following a single dose of 500 mg, the apparent terminal elimination half-life of azithromycin is 68 hours.[35] Biliary excretion of azithromycin, predominantly unchanged, is a major route of elimination. Over the course of a week, about 6% of the administered dose appears as unchanged drug in urine.
History
A team of researchers at the pharmaceutical company Pliva in Zagreb, SR Croatia, Yugoslavia, — Gabrijela Kobrehel, Gorjana Radobolja-Lazarevski, and Zrinka Tamburašev, led by Dr. Slobodan Đokić — discovered azithromycin in 1980.[6] It was patented in 1981. In 1986, Pliva and Pfizer signed a licensing agreement, which gave Pfizer exclusive rights for the sale of azithromycin in Western Europe and the United States. Pliva put its azithromycin on the market in Central and Eastern Europe under the brand name Sumamed in 1988. Pfizer launched azithromycin under Pliva’s license in other markets under the brand name Zithromax in 1991.[36] Patent protection ended in 2005.[37]
Society and culture
Zithromax (azithromycin) 250 mg tablets (CA)
Cost
It is available as a generic medication.[9] The wholesale cost is about US$0.18 to US$2.98 per dose.[10] In the United States it is about US$4 for a course of treatment as of 2018.[11] In India, it is about US$1.70 for a course of treatment.[citation needed]
Available forms
Azithromycin is commonly administered in film-coated tablet, capsule, oral suspension, intravenous injection, granules for suspension in sachet, and ophthalmic solution.[2]
Usage
In 2010, azithromycin was the most prescribed antibiotic for outpatients in the US,[38] whereas in Sweden, where outpatient antibiotic use is a third as prevalent, macrolides are only on 3% of prescriptions.[39]
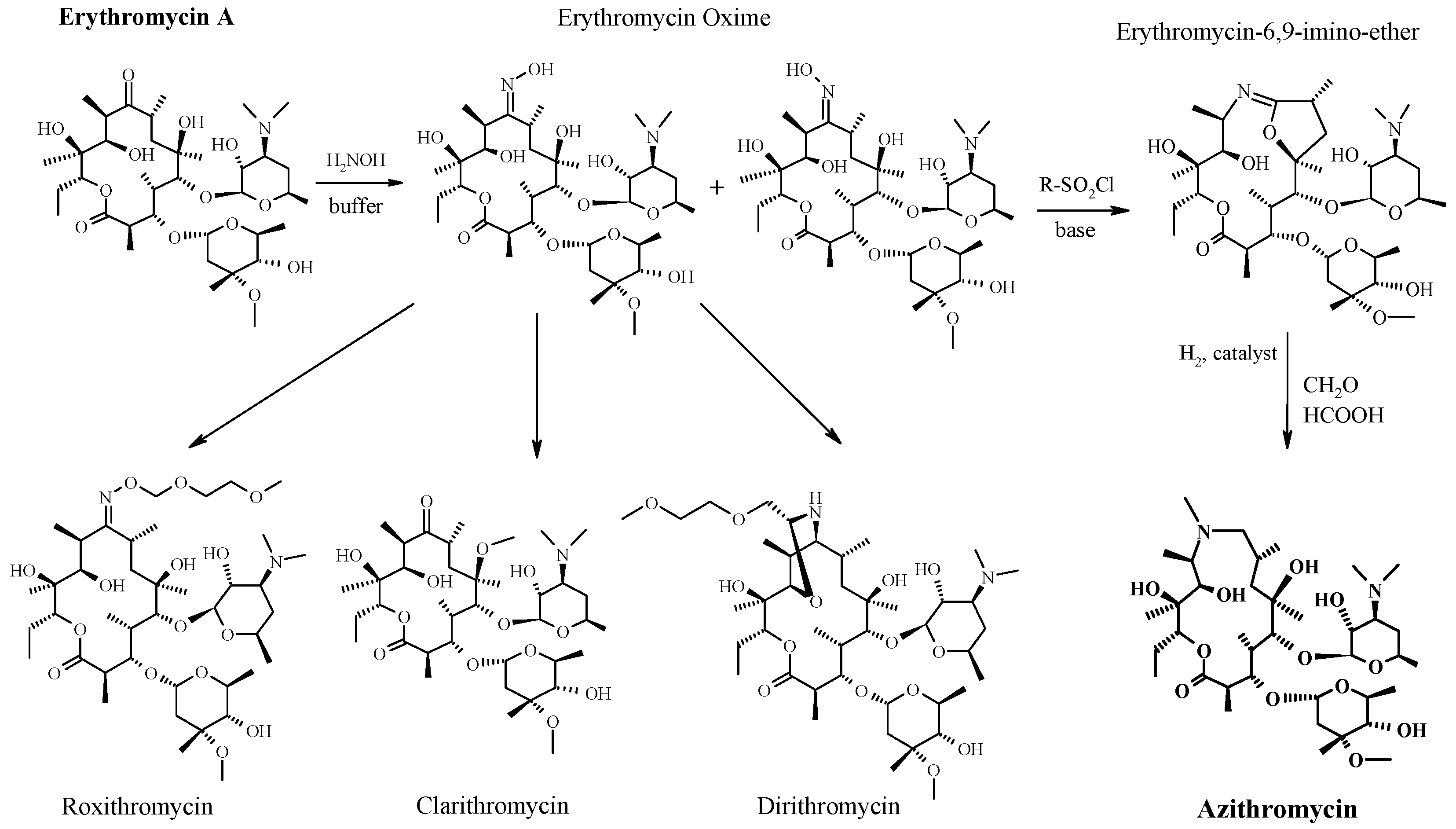
READ
References
- ^ Jump up to:ab “Azithromycin Use During Pregnancy”. Drugs.com. 2 May 2019. Retrieved 24 December 2019.
- ^ Jump up to:abcdef “Azithromycin International Brands”. Drugs.com. Archived from the original on 28 February 2017. Retrieved 27 February 2017.
- ^ Jump up to:abcdefghijklm “Azithromycin”. The American Society of Health-System Pharmacists. Archived from the original on 5 September 2015. Retrieved 1 August 2015.
- ^ Jump up to:ab “Azithromycin use while Breastfeeding”. Archived from the original on 5 September 2015. Retrieved 4 September 2015.
- ^ Greenwood, David (2008). Antimicrobial drugs : chronicle of a twentieth century medical triumph (1. publ. ed.). Oxford: Oxford University Press. p. 239. ISBN9780199534845. Archived from the original on 5 March 2016.
- ^ Jump up to:ab Fischer, Jnos; Ganellin, C. Robin (2006). Analogue-based Drug Discovery. John Wiley & Sons. p. 498. ISBN9783527607495.
- ^ World Health Organization (2019). World Health Organization model list of essential medicines: 21st list 2019. Geneva: World Health Organization. hdl:10665/325771. WHO/MVP/EMP/IAU/2019.06. License: CC BY-NC-SA 3.0 IGO.
- ^ World Health Organization (2019). Critically important antimicrobials for human medicine (6th revision ed.). Geneva: World Health Organization. hdl:10665/312266. ISBN9789241515528. License: CC BY-NC-SA 3.0 IGO.
- ^ Jump up to:ab Hamilton, Richart (2015). Tarascon Pocket Pharmacopoeia 2015 Deluxe Lab-Coat Edition. Jones & Bartlett Learning. ISBN9781284057560.
- ^ Jump up to:ab “Azithromycin”. International Drug Price Indicator Guide. Retrieved 4 September 2015.
- ^ Jump up to:ab “NADAC as of 2018-05-23”. Centers for Medicare and Medicaid Services. Retrieved 24 May 2018.
- ^ “The Top 300 of 2019”. clincalc.com. Retrieved 22 December2018.
- ^ Taylor SP, Sellers E, Taylor BT (2015). “Azithromycin for the Prevention of COPD Exacerbations: The Good, Bad, and Ugly”. Am. J. Med. 128 (12): 1362.e1–6. doi:10.1016/j.amjmed.2015.07.032. PMID26291905.
- ^ Mandell LA, Wunderink RG, Anzueto A, Bartlett JG, Campbell GD, Dean NC, Dowell SF, File TM, Musher DM, Niederman MS, Torres A, Whitney CG (2007). “Infectious Diseases Society of America/American Thoracic Society consensus guidelines on the management of community-acquired pneumonia in adults”. Clin. Infect. Dis. 44 Suppl 2: S27–72. doi:10.1086/511159. PMID17278083.
- ^ Jump up to:ab “Gonococcal Infections – 2015 STD Treatment Guidelines”. Archived from the original on 1 March 2016.
- ^ Burton M, Habtamu E, Ho D, Gower EW (2015). “Interventions for trachoma trichiasis”. Cochrane Database Syst Rev. 11 (11): CD004008. doi:10.1002/14651858.CD004008.pub3. PMC4661324. PMID26568232.
- ^ Rosenfeld RM, Piccirillo JF, Chandrasekhar SS, Brook I, Ashok Kumar K, Kramper M, Orlandi RR, Palmer JN, Patel ZM, Peters A, Walsh SA, Corrigan MD (2015). “Clinical practice guideline (update): adult sinusitis”. Otolaryngol Head Neck Surg. 152 (2 Suppl): S1–S39. doi:10.1177/0194599815572097. PMID25832968.
- ^ Hauk L (2014). “AAP releases guideline on diagnosis and management of acute bacterial sinusitis in children one to 18 years of age”. Am Fam Physician. 89 (8): 676–81. PMID24784128.
- ^ Neff MJ (2004). “AAP, AAFP release guideline on diagnosis and management of acute otitis media”. Am Fam Physician. 69 (11): 2713–5. PMID15202704.
- ^ Randel A (2013). “IDSA Updates Guideline for Managing Group A Streptococcal Pharyngitis”. Am Fam Physician. 88 (5): 338–40. PMID24010402.
- ^ The Guardian newspaper: ‘Super-gonorrhoea’ outbreak in Leeds, 18 September 2015Archived 18 September 2015 at the Wayback Machine
- ^ Lippincott Illustrated Reviews : Pharmacology Sixth Edition. p. 506.
- ^ Jump up to:ab “US azithromycin label”(PDF). FDA. February 2016. Archived(PDF) from the original on 23 November 2016.
- ^ Simoens, Steven; Laekeman, Gert; Decramer, Marc (May 2013). “Preventing COPD exacerbations with macrolides: A review and budget impact analysis”. Respiratory Medicine. 107 (5): 637–648. doi:10.1016/j.rmed.2012.12.019. PMID23352223.
- ^ Gotfried, Mark H. (February 2004). “Macrolides for the Treatment of Chronic Sinusitis, Asthma, and COPD”. CHEST. 125 (2): 52S–61S. doi:10.1378/chest.125.2_suppl.52S. ISSN0012-3692. PMID14872001.
- ^ Zarogoulidis, P.; Papanas, N.; Kioumis, I.; Chatzaki, E.; Maltezos, E.; Zarogoulidis, K. (May 2012). “Macrolides: from in vitro anti-inflammatory and immunomodulatory properties to clinical practice in respiratory diseases”. European Journal of Clinical Pharmacology. 68 (5): 479–503. doi:10.1007/s00228-011-1161-x. ISSN1432-1041. PMID22105373.
- ^ Steel, Helen C.; Theron, Annette J.; Cockeran, Riana; Anderson, Ronald; Feldman, Charles (2012). “Pathogen- and Host-Directed Anti-Inflammatory Activities of Macrolide Antibiotics”. Mediators of Inflammation. 2012: 584262. doi:10.1155/2012/584262. PMC3388425. PMID22778497.
- ^ Mori F, Pecorari L, Pantano S, Rossi M, Pucci N, De Martino M, Novembre E (2014). “Azithromycin anaphylaxis in children”. Int J Immunopathol Pharmacol. 27 (1): 121–6. doi:10.1177/039463201402700116. PMID24674687.
- ^ Dart, Richard C. (2004). Medical Toxology. Lippincott Williams & Wilkins. p. 23.
- ^ Tilelli, John A.; Smith, Kathleen M.; Pettignano, Robert (2006). “Life-Threatening Bradyarrhythmia After Massive Azithromycin Overdose”. Pharmacotherapy. 26 (1): 147–50. doi:10.1592/phco.2006.26.1.147. PMID16506357.
- ^ Baselt, R. (2008). Disposition of Toxic Drugs and Chemicals in Man (8th ed.). Foster City, CA: Biomedical Publications. pp. 132–133.
- ^ Denise Grady (16 May 2012). “Popular Antibiotic May Raise Risk of Sudden Death”. The New York Times. Archived from the original on 17 May 2012. Retrieved 18 May 2012.
- ^ Ray, Wayne A.; Murray, Katherine T.; Hall, Kathi; Arbogast, Patrick G.; Stein, C. Michael (2012). “Azithromycin and the Risk of Cardiovascular Death”. New England Journal of Medicine. 366(20): 1881–90. doi:10.1056/NEJMoa1003833. PMC3374857. PMID22591294.
- ^ “FDA Drug Safety Communication: Azithromycin (Zithromax or Zmax) and the risk of potentially fatal heart rhythms”. FDA. 12 March 2013. Archived from the original on 27 October 2016.
- ^ Jump up to:ab “Archived copy”. Archived from the original on 14 October 2014. Retrieved 10 October 2014.
- ^ Banić Tomišić, Z. (2011). “The Story of Azithromycin”. Kemija U Industriji. 60 (12): 603–617. ISSN0022-9830. Archived from the original on 8 September 2017.
- ^ “Azithromycin: A world best-selling Antibiotic”. http://www.wipo.int. World Intellectual Property Organization. Retrieved 18 June 2019.
- ^ Hicks, LA; Taylor TH, Jr; Hunkler, RJ (April 2013). “U.S. outpatient antibiotic prescribing, 2010”. The New England Journal of Medicine. 368 (15): 1461–1462. doi:10.1056/NEJMc1212055. PMID23574140.
- ^ Hicks, LA; Taylor TH, Jr; Hunkler, RJ (September 2013). “More on U.S. outpatient antibiotic prescribing, 2010”. The New England Journal of Medicine. 369 (12): 1175–1176. doi:10.1056/NEJMc1306863. PMID24047077.
External links
Keywords: Antibacterial (Antibiotics); Macrolides.
- “Azithromycin”. Drug Information Portal. U.S. National Library of Medicine.
![]() |
|
![]() |
|
Clinical data | |
---|---|
Trade names | Zithromax, Azithrocin, others[2] |
Other names | 9-deoxy-9α-aza-9α-methyl-9α-homoerythromycin A |
AHFS/Drugs.com | Monograph |
MedlinePlus | a697037 |
License data |
|
Pregnancy category |
|
Routes of administration |
By mouth (capsule, tablet or suspension), intravenous, eye drop |
Drug class | Macrolide antibiotic |
ATC code | |
Legal status | |
Legal status |
|
Pharmacokinetic data | |
Bioavailability | 38% for 250 mg capsules |
Metabolism | Liver |
Elimination half-life | 11–14 h (single dose) 68 h (multiple dosing) |
Excretion | Biliary, kidney (4.5%) |
Identifiers | |
CAS Number | |
PubChem CID | |
IUPHAR/BPS | |
DrugBank | |
ChemSpider | |
UNII | |
KEGG | |
ChEBI | |
ChEMBL | |
NIAID ChemDB | |
CompTox Dashboard (EPA) | |
ECHA InfoCard | 100.126.551 ![]() |
Chemical and physical data | |
Formula | C38H72N2O12 |
Molar mass | 748.984 g·mol−1 g·mol−1 |
3D model (JSmol) | |
/////////AZITHROMYCIN, Antibacterial, Antibiotics, Macrolides, CORONA VIRUS, COVID 19, アジスロマイシン ,
Substances Referenced in Synthesis Path
CAS-RN Formula Chemical Name CAS Index Name
76801-85-9 C37H70N2O12 2-deoxo-9a-aza-9a-homoerythromycin A 1-Oxa-6-azacyclopentadecan-15-one,
13-[(2,6-dideoxy-3-C-methyl-3-O-methyl-α-L-ribo-hexopyranosyl)oxy]-2-eth- yl-3,4,10-trihydroxy-3,5,8,10,12,14-hexamethyl-11-[[3,4,6-trideoxy-3-(dimethylamino)-β-D-xylo-hexopyranosyl]oxy]-, [2R-(2
R*,3S*,4R*,5R*,8R*,10R*,11R*,12S*,13S*,1
4R*)]-
90503-04-1 C37H70N2O14 [2R-(2R*,3S*,4R*,5R*,8R*,10R*,11R*,12S*,
13S*,14R*)]-13-[(2,6-dideoxy-3-C-methyl3-O-methyl-α-L-ribo-hexopyranosyl)
oxy]-2-ethyl-3,4,6,10-tetrahydroxy3,5,8,10,12,14-hexamethyl-13-[[3,4,6-
trideoxy-3-(dimethyloxidoamino)-
β-D-xylo-hexopyranosyl] oxy]-1-oxa-6-azacyclopentadecan-15-one
1-Oxa-6-azacyclopentadecan-15-one,
13-[(2,6-dideoxy-3-C-methyl-3-Omethyl-α-L-ribo-hexopyranosyl)
oxy]-2-ethyl-3,4,6,10-tetrahydroxy3,5,8,10,12,14-hexamethyl-13-[[3,4,6-
trideoxy-3-(dimethyloxidoamino)-β-Dxylo-hexopyranosyl]oxy]-, [2R-(2R*,3S*,4R
*,5R*,8R*,10R*,11R*,12S*,13S*,14R*)]-
90503-05-2 C38H72N2O14 [2R-(2R*,3S*,4R*,5R*,8R*,10R*,11R*,12S*,
13S*,14R*)]-13-[(2,6-dideoxy-3-C-methyl3-O-methyl-α-L-ribo-hexopyranosyl) oxy]-2-ethyl-3,4,10-trihydroxy3,5,6,8,10,12,14-heptamethyl-11-[[3,4,6-
trideoxy-3-(dimethyloxidoamino)-
β-D-xylo-hexopyranosyl]
oxy]-1-oxa-6-azacyclopentadecan-15-one
6-oxide
1-Oxa-6-azacyclopentadecan-15-one,
13-[(2,6-dideoxy-3-C-methyl-3-Omethyl-α-L-ribo-hexopyranosyl)
oxy]-2-ethyl-3,4,10-trihydroxy3,5,6,8,10,12,14-heptamethyl-11-[[3,4,6-
trideoxy-3-(dimethyloxidoamino)-βD-xylo-hexopyranosyl]oxy]-, 6-oxide,
[2R-(2R*,3S*,4R*,5R*,8R*,10R*,11R*,12S*,1
3S*,14R*)]-
50-00-0 CH2O formaldehyde Formaldehyde
74-88-4 CH3I methyl iodide Methane, iodoTrade Names
Country Trade Name Vendor Annotation
D Ultreon Pfizer
Zithromax Pfizer Pharma/Gödecke/Parke-Davis
numerous generic preparations
F Azadose Pfizer
Monodose Pfizer
Zithromax Pfizer
GB Zithromax Pfizer
I Azitrocin Bioindustria
Ribotrex Pierre Fabre
Trocozina Sigma-Tau
Zithromax Pfizer
J Zithromac Pfizer
USA Azasite InSite Vision
Zithromax Pfizer as dihydrate
Formulations
cps. 100 mg, 250 mg; Gran. 10%; susp. 200 mg (as dihydrate); tabl. 250 mg
References
Djokic, S. et al.: J. Antibiot. (JANTAJ) 40, 1006 (1987).
a DOS 3 140 449 (Pliva; appl. 12.10.1981; YU-prior. 6.3.1981).
US 4 517 359 (Pliva; 14.5.1985; appl. 22.9.1981; YU-prior. 6.3.1981).
b EP 101 186 (Pliva; appl. 14.7.1983; USA-prior. 19.7.1982, 15.11.1982).
US 4 474 768 (Pfizer; 2.10.1984; prior. 19.7.1982, 15.11.1982).
educt by ring expansion of erythromycin A oxime by Beckmann rearrangement:
Djokic, S. et al.: J. Chem. Soc., Perkin Trans. 1 (JCPRB4) 1986, 1881-1890.
Bright, G.M. et al.: J. Antibiot. (JANTAJ) 41, 1029 (1988). US 4 328 334 (Pliva; 4.5.1982; YU-prior. 2.4.1979).
stable, non-hygroscopic dihydrate: EP 298 650 (Pfizer; appl. 28.6.1988).
medical use for treatment of protozoal infections:
US 4 963 531 (Pfizer; 16.10.1990; prior. 16.8.1988, 10.9.1987).
Molnupiravir, EIDD 2801
EIDD 2801
Molecular Formula: | C13H19N3O7 |
---|---|
Molecular Weight: | 329.31 g/mol |
[(2R,3S,4R,5R)-3,4-dihydroxy-5-[4-(hydroxyamino)-2-oxopyrimidin-1-yl]oxolan-2-yl]methyl 2-methylpropanoate
UNII YA84KI1VEW
CAS 2349386-89-4
Molnupiravir (development codes MK-4482 and EIDD-2801) is an experimental antiviral drug which is orally active (can be taken orally) and was developed for the treatment of influenza. It is a prodrug of the synthetic nucleoside derivative N4-hydroxycytidine, and exerts its antiviral action through introduction of copying errors during viral RNA replication.[1][2] Activity has also been demonstrated against coronaviruses including SARS, MERS and SARS-CoV-2.[3]
The drug was developed at Emory University by the university’s drug innovation company, Drug Innovation Ventures at Emory (DRIVE). It was then acquired by Miami-based company Ridgeback Biotherapeutics, who later partnered with Merck & Co. to develop the drug further.
Safety Controversy
In April 2020, a whistleblower complaint by former Head of US Biomedical Advanced Research and Development Authority (BARDA) Rick Bright revealed concerns over providing funding for the further development of molnupiravir due to similar drugs having mutagenic properties (producing birth defects).[4] A previous company, Pharmasset, that had investigated the drug’s active ingredient had abandoned it. These claims were denied by George Painter, CEO of DRIVE, noting that toxicity studies on molnupiravir had been carried out and data provided to regulators in the US and UK, who permitted safety studies in humans to move forward in the spring of 2020. Also at this time, DRIVE and Ridgeback Biotherapeutics stated they planned future safety studies in animals.[5]
COVID-19
After being found to be active against SARS-CoV-2 in March 2020, molnupiravir was tested in a preliminary human study for “Safety, Tolerability, and Pharmacokinetics” in healthy volunteers in the UK and US.[6] In June 2020, Ridgeback Biotherapeutics announced it was moving to Phase II trials to test the efficacy of the drug as a treatment for COVID-19.[7] Two trials of small numbers of hospitalized and non-hospitalized patients in the US and the UK were underway in July.[8][9] In late July 2020, and without yet releasing any medical data, Merck, which had been partnering with Ridgeback Biotherapeutics on developing the drug, announced its intention to move molnupiravir to late stage trials beginning in September 2020.[10] On October 19 2020, Merck began a one year Stage 2/3 trial focused on hospitalized patients.[11]

join me on Linkedin
Anthony Melvin Crasto Ph.D – India | LinkedIn
join me on Researchgate
RESEARCHGATE

join me on Facebook
Anthony Melvin Crasto Dr. | Facebook
join me on twitter
Anthony Melvin Crasto Dr. | twitter
+919321316780 call whatsaapp
EMAIL. amcrasto@gmail.com
PATENT
WO 2019113462
https://patentscope.wipo.int/search/en/detail.jsf?docId=WO2019113462
Example 10: Synthesis of EIDD-2801
A 1L round bottom flask was charged with uridine (25 g, 102.38 mmol) and acetone (700 mL). The reaction mixture was allowed to stir at rt. The slurry was then treated with sulfuric acid (0.27 mL, 5.12 mmol). Stirring was allowed to continue at rt for 18 hours. The reaction was quenched with 100 mL of trimethylamine and was used in the next step without further pruficication.
A 1L round bottom flask was charged with the reaction mixture from the previous reaction. Triethylamine (71.09 mL, 510.08 mmol) and 4-dimethylaminopyridine (0.62 g, 5.1 mmol) were then added. The flask was cooled using an ice bath and then 2-methylpropanoyl 2-methylpropanoate (17.75 g, 112.22 mmol) was slowly added. The reaction mixture was allowed to stir at rt until the reaction was complete. The reaction mixture was concentrated under reduced pressure, and the residue was dissolved in 600 mL ethyl acetate and washed with saturated aqueous bicarbonate solution x 2, water x 2 and brine x 2. The organics were dried over sodium sulfate and concentrated under reduced pressure to yield a clear colorless oil. The crude product was used in the next step without further purification.
A 1L round bottom flask was charged with the crude product from above (36 g, 101.59 mmol) and MeCN (406.37 mL). The reaction mixture was allowed to stir until all the starting material was dissolved. Next, 1,2, 4-triazole (50.52 g, 731.46 mmol) was added followed by the addition of N,N-diethylethanamine (113.28 mL, 812.73 mmol). The reaction mixture was allowed to stir at rt until all solids dissolved. The reaction was then cooled to 0°C using an ice bath. Phosphorous oxychloride (24.44 mL, 152.39 mmol) was added slowly. The slurry that formed was allowed to stir under argon while slowly warming to rt. The reaction was then allowed to stir until complete by TLC (EtOAc). The reaction was then quenched by the addition of lOOmL of water. The slurry then became a dark colored solution, which was
then concentrated under reduced pressure. The residue was dissolved in DCM and washed with water and brine. The organics were then dried over sodium sulfate, filtered, and concentrated under reduced pressure. The product was purified by silica gel chromatography (2 x 330 g columns). All fractions containing product were collected and concentrated under reduced pressure.
A 500 mL round bottom flask was charged with the product from the previous step (11.8 g, 29.11 mmol) and isopropyl alcohol (150 mL). The reaction mixture was allowed to stir at rt until all solids dissolved. Next, hydroxylamine (1.34 mL, 43.66 mmol) was added and stirring continued at ambient temperature. When the reaction was complete (HPLC) some solvent was removed under high vacuum at ambient temperature. The remaining solvent was removed under reduced pressure at 45°C. The resulting residue was dissolved in EtOAc and was washed with water and brine. The organics were dried over sodium sulfate, filtered, and concentrated under reduced pressure to yield oil. Crystals formed upon standing at rt. The crystals were collected by filtration, washed with ether x 3, and dried in vacuo to provide the product as a white solid.
A 200 mL round bottom flask was charged with the product from the previous step (6.5 g, 17.6 mmol) and formic acid (100 mL, 2085.6 mmol). The reaction mixture was allowed to stir at rt overnight. The progress of the reaction was monitored by HPLC. The reaction mixture was concentrated under reduced pressure at 42°C to yield a clear, pale pink oil. Next, 30 mL of ethanol was added. Solvent was then removed under reduced pressure. MTBE (50 mL) was added to the solid and heated. Next, isopropyl alcohol was added and heating was continued until all solid material dissolved (5 mL). The solution was then allowed to cool and stand at rt.
A solid started to form after about lhr. The solids were collected by filtration, washed with MTBE, and dried in vacuo to yield the EIDD-2801 as a white solid. The filtrate was concentrated under reduced pressure to yield a sticky solid, which was dissolved in a small amount of isopropyl alcohol with heating. The solution was allowed to stand at rt overnight. A solid formed in the flask, which was collected by filtration, rinsed with isopropyl alcohol and MTBE, and dried in vacuo to an additional crop of desired product.
EIDD-2801 (25 g) was dissolved in 250 mL of isopropyl alcohol by heating to 70°C to give a clear solution. The warm solution was polish filtered and filtrate transferred to 2L three neck flask with overhead stirrer. It was warmed back to 70°C and MTBE (250 mL) was slowly added into the flask. The clear solution was seeded and allowed to cool slowly to rt with stirring for 18 hrs. The EIDD-2801 solid that formed was filtered and washed with MTBE and dried at 50°C under vacuum for l8hours. The filtrate was concentrated, redissolved in 50 mL isopropyl alcohol and 40 mL MTBE by warming to give clear solution and allowed to stand at rt to give a second crop of EIDD-2801.
Example 11: General synthesis for Deuteration
389 390
The lactone 389 (0.0325 mol) was added to a dry flask under an argon atmosphere and was then dissolved in dry THF (250 mL). The solution as then cooled to -78°C and a DIBAL-D solution in toluene (0.065 mol) was dropwise. The reaction was allowed to stir at -78°C for 3-4 hours. The reaction was then quenched with the slow addition of water (3 mL). The reaction was then allowed to stir while warming to rt. The mixture was then diluted with two volumes of diethyl ether and was then poured into an equal volume of saturated sodium potassium tartrate solution. The organic layer was separated, dried over MgSCri. filtered, and concentrated under reduced pressure. The residue was purified on silica eluting with hexanes/ethyl acetate. The resulting lactol 390 was then converted to an acetate or benzolyate and subjected to cytosine coupling conditions and then further elaborated to N-hydroxycytidine.
PATENT
WO 2019173602
https://patentscope.wipo.int/search/en/detail.jsf?docId=WO2019173602
PAPER
ChemRxiv (2020), 1-3.
AND
ChemRxiv (2020), 1-2
PAPER
A Concise Route to MK-4482 (EIDD-2801) from Cytidine: Part 2
Synlett (2020), Ahead of Print.
A new route to MK-4482 was developed. The route replaces uridine with the more available and less expensive cytidine. Low-cost, simple reagents are used for the chemical transformations, and the yield is improved from 17% to 44%. A step is removed from the longest linear sequence, and these advancements are expected to expand access to MK-4482 should it become a viable drug substance.
To a 20 mL vial was added N-hydroxycytidine acetonide ester 5 (0.25 g, 96% purity) followed by formic acid (4 mL). The resultant solution was stirred at room temperature for 4 h 20 min. Solvent was removed under reduced pressure and fresh EtOH (5 mL) was added. The resultant solution was again concentrated under vacuum to afford an oil. Methyl tert-butyl ether and IPA (5 mL each) were successively added as described earlier for preparation of compound 4 and concentrated to give 0.205 g of crude material (77% assay yield, 79% purity). This material was purified by silica gel column chromatography in 8 % MeOH/ Chloroform to afford 130 mg of EIDD-2801 as a solid (60% isolated yield corrected for purity, 98% purity) 1H NMR (600 MHz, CD3OD): δ 6.91 (d, J = 8.2 Hz, 1H), 5.82 (d, J = 4.8 Hz, 1H), 5.61 (d, J = 8.2 Hz, 1H), 4.29 (d, J = 3.6 Hz, 2H), 4.14 (t, J = 4.9 Hz, 1H), 4.08 (p, J = 4.9 Hz, 2H), 2.62 (septet, J = 7.0 Hz, 1H), 1.19 (d, J = 7.0 Hz, 6H); 13C NMR (151 MHz, CD3OD): δ 178.6, 151.81, 146.44, 132.04, 99.84, 90.74, 82.88, 74.67, 71.80, 65.23, 35.45, 27.49, 19.65, 19.61.
One-Pot Transamination/Deprotection of 4 to EIDD-2801: To acetonide ester 4 (1.03 g, 77% Purity) in a 100 mL single neck round bottom flask was added hydroxylamine sulfate (1.09 g, 3.2 equiv.) followed by 40% IPA (20 mL prepared by mixing 12 mL of water and 8 mL of 99.5% IPA. The resultant solution was heated to 78˚C (internal temperature 72-73 ˚C) for 23 h upon which time HPLC showed the formation of EIDD-2801. Solvent was removed on a rotary evaporator and isopropanol (20 mL) was then added. The resulting slurry was sonicated for 5 minutes. The insoluble residue was then filtered and the filtrate concentrated under reduced pressure to afford crude material. (1.34 g, 38% purity, 69% assay yield). The resultant material was purified by silica gel chromatography (5-6% MeOH/DCM) to provide pure EIDD-2801 as two fractions (0.26 g, >99% purity, 36% corrected yield) as an yellow solid and 0.27 g (69.5% purity, 26% corrected yield) as a pinkish solid. The lower purity material was subjected to a second column purification again using 7% MeOH/ DCM to afford 0.137 g of material with 90% purity by NMR. The combined yield thus was estimated to be 53%. The 1H NMR spectrum of the product thus obtained matched the one obtained in the sequential approach as outlined above.
SYN
- A High‐Yielding Synthesis of EIDD‐2801 from Uridine,
Alexander Steiner, Desiree Znidar, Sándor B. Ötvös, David R. Snead, Doris Dallinger, C. Oliver Kappe,
Eur. J. Org. Chem. 2020.
https://doi.org/10.1002/ejoc.202001340
EIDD-2801 was isolated in 69% yield (307 mg) and ≥99% purity as a white
solid.
1H-NMR (300 MHz, MeOH-d4) δ 6.91 (d, J= 8.3 Hz, 1H), 5.82 (d, J= 4.8 Hz, 1H), 5.61 (d, J= 8.2 Hz, 1H), 4.29
(d, J= 3.6 Hz, 2H), 4.15-4.07 (m, 3H), 2.62 (sept, J= 7.0 Hz, 1H), 1.18 (d, J= 7.0 Hz 6H);
13C-NMR (75 MHz,
MeOH-d4Ϳ δ 178.2, 151.5, 146.1, 131.7, 99.5, 90.4, 82.5, 74.3, 71.5, 64.9, 35.1, 19.3, 19.3. The NMR data
is in agreement with previously published values.[2] HRMS (ESI, positive mode): m/z [M + H]+
Calcd for
[C13H20N3O7 +H]+
: 330.1296, found: 330.1297.
SYN
C. Oliver Kappe, Doris Dallinger, University of Graz, Austria, and colleagues have developed an improved synthesis of EIDD-2801 from uridine (pictured below) by strategically reordering the synthetic steps. The reaction sequence starts with the activation of uridine with 1,2,4-triazole and continues with a telescoped acetonide protection/esterification and a telescoped hydroxyamination/acetonide deprotection. Telescoped reaction sequences consist of two or more than one one-pot procedures that are performed back-to-back without a work-up step in-between. A continuous flow process was used for the final acetonide deprotection, which improved selectivity and reproducibility.
SYN
https://www.frontiersin.org/articles/10.3389/fphar.2020.01013/full
SYN
http://www.rsc.org/suppdata/d0/cc/d0cc05944g/d0cc05944g1.pdf
To a solution of 5’-O-isobutyrylcytidine 4 (1.0 g, 90% purity, 2.87 mmol, 1.0 eq) in 2-propanol (15 ml), hydroxylamine sulphate (2.12 g, 12.93 mmol, 4.5 eq.) was added and reaction was stirred for 20 h at 78 C. Upon completion, the reaction was cooled to room temperature. The organic layer (upper layer) was separated from biphasic reaction mixture. The aqueous layer was washed with 2-propanol (2 X 5 mL). The combined organic layer was concentrated using rotary evaporation and the crude was purified by column chromatography with a gradient of 2-15% methanol in dichloromethane to yield EIDD-2801 (1) as a white solid (963 mg, 94% purity, 96% yield). 1H NMR (600 MHz, D2O) δ 6.98 (d, J = 8.3 Hz, 1H), 5.87 (d, J = 5.0 Hz, 1H), 5.78 (d, J = 8.2 Hz, 1H), 4.39 – 4.33 (m, 3H), 4.28 (dd, J = 6.6, 3.4 Hz, 2H), 2.69 (hept, J = 7.0 Hz, 1H), 1.17 (d, J = 3.7 Hz, 3H), 1.16 (d, J = 3.7 Hz, 3H). 13C NMR (126 MHz, D2O) δ 18.1, 18.2, 33.9, 48.8, 63.6, 69.6, 72.5, 81.0, 88.5, 98.8, 131.1, 151.1, 179.8 ppm; LRMS: 330.1 [M+H]+ ; HRMS (ESI): calcd. for C13H19N3O7 [M+H]+ 330.1296, found 330.1302; Purity: 94% (assessed by qNMR).
https://pubs.rsc.org/en/content/articlehtml/2020/cc/d0cc05944g
![]() |
||
Fig. 2 A new route to MK-4482 from cytidine. |
References
- ^ Toots M, Yoon JJ, Cox RM, Hart M, Sticher ZM, Makhsous N, et al. (October 2019). “Characterization of orally efficacious influenza drug with high resistance barrier in ferrets and human airway epithelia”. Science Translational Medicine. 11 (515): eaax5866. doi:10.1126/scitranslmed.aax5866. PMC 6848974. PMID 31645453.
- ^ Toots M, Yoon JJ, Hart M, Natchus MG, Painter GR, Plemper RK (April 2020). “Quantitative efficacy paradigms of the influenza clinical drug candidate EIDD-2801 in the ferret model”. Translational Research. 218: 16–28. doi:10.1016/j.trsl.2019.12.002. PMID 31945316.
- ^ Sheahan TP, Sims AC, Zhou S, Graham RL, Pruijssers AJ, Agostini ML, et al. (April 2020). “An orally bioavailable broad-spectrum antiviral inhibits SARS-CoV-2 in human airway epithelial cell cultures and multiple coronaviruses in mice”. Science Translational Medicine. 12 (541): eabb5883. doi:10.1126/scitranslmed.abb5883. PMC 7164393. PMID 32253226.
- ^ Halford, Bethany. “An emerging antiviral takes aim at COVID-19”. Retrieved 1 August 2020.
- ^ Cohen, Jon; Piller, Charles (13 May 2020). “Emails offer look into whistleblower charges of cronyism behind potential COVID-19 drug”. Science. Retrieved 1 August 2020.
- ^ “COVID-19 First In Human Study to Evaluate Safety, Tolerability, and Pharmacokinetics of EIDD-2801 in Healthy Volunteers”. ClinicalTrials.gov. Retrieved 1 June 2020.
- ^ “Ridgeback Biotherapeutics Announces Launch of Phase 2 Trials Testing EIDD-2801 as Potential Treatment for COVID-19”. Business Wire. Retrieved 4 July 2020.
- ^ “A Safety, Tolerability and Efficacy of EIDD-2801 to Eliminate Infectious Virus Detection in Persons With COVID-19”. ClinicalTrials.gov. Retrieved 4 July 2020.
- ^ “The Effect of EIDD-2801 on Viral Shedding of SARS-CoV-2 (COVID-19)”. ClinicalTrials.gov. Retrieved 4 July 2020.
- ^ Court, Emma (31 July 2020). “Merck pushes ahead on COVID-19 treatment, vaccines”. Retrieved 31 July 2020.
- ^ ClinicaL trials register : Efficacy and Safety of Molnupiravir (MK-4482) in Hospitalized Adult Participants With COVID-19 (MK-4482-001)
Electron microscope image of SARS virus in a tissue culture isolate, courtesy of CDC Public Health Image Library.
The drug EIDD-1931 was effective against SARS and MERS viruses in the laboratory, and a modified version (EIDD-2801) could potentially be valuable against 2019-nCoV.
https://news.emory.edu/stories/2020/02/coronavirus_eidd/index.html
Emory, collaborators testing antiviral drug as potential treatment for coronaviruses
An antiviral compound discovered at Emory University could potentially be used to treat the new coronavirus associated with the outbreak in China and spreading around the globe. Drug Innovation Ventures at Emory (DRIVE), a non-profit LLC wholly owned by Emory, is developing the compound, designated EIDD-2801.
In testing with collaborators at the University of North Carolina at Chapel Hill and Vanderbilt University Medical Center, the active form of EIDD-2801, which is called EIDD-1931, has shown efficacy against the related coronaviruses SARS (Severe Acute Respiratory Syndrome)- and MERS-CoV (Middle East Respiratory Syndrome Coronavirus). Some of the data was recently published in Journal of Virology.
EIDD-2801 is an oral ribonucleoside analog that inhibits the replication of multiple RNA viruses, including respiratory syncytial virus, influenza, chikungunya, Ebola, Venezuelan equine encephalitis virus, and Eastern equine encephalitis viruses.
“We have been planning to enter human clinical tests of EIDD-2801 for the treatment of influenza, and recognized that it has potential activity against the current novel coronavirus,” says George Painter, PhD, director of the Emory Institute for Drug Development (EIDD) and CEO of DRIVE. “Based on the drug’s broad-spectrum activity against viruses including influenza, Ebola and SARS-CoV/MERS-CoV, we believe it will be an excellent candidate.”
“Our studies in the Journal of Virology show potent activity of the EIDD-2801 parent compound against multiple coronaviruses including SARS and MERS,” says Mark Denison, MD, the Stahlman Professor of Pediatrics and director of pediatric infectious diseases at Vanderbilt University School of Medicine. “It also has a strong genetic barrier to development of viral resistance, and its oral bioavailability makes it a candidate for use during an outbreak.”
“Generally speaking, seasonal flu is still a much more common threat than this coronavirus, however, novel emerging coronaviruses represent a considerable threat to global health as evidenced by the new 2019-nCoV,” said Ralph Baric, PhD, an epidemiology professor at the University of North Carolina’s Gillings School of Global Public Health. “But the reason the new coronavirus is so concerning is that it’s much more likely to be deadly than the flu – fatal for about one in 25 people versus one in 1,000 for the flu.”
The development of EIDD-2801 has been funded in whole or in part with Federal funds from the National Institute of Allergy and Infectious Diseases (NIAID), under contract numbers HHSN272201500008C and 75N93019C00058, and from the Defense Threat Reduction Agency (DTRA), under contract numbers HDTRA1-13-C-0072 and HDTRA1-15-C-0075, for the treatment of Influenza, coronavirus, chikungunya, and Venezuelan equine encephalitis virus.
About DRIVE: DRIVE is a non-profit LLC wholly owned by Emory started as an innovative approach to drug development. Operating like an early stage biotechnology company, DRIVE applies focus and industry development expertise to efficiently translate discoveries to address viruses of global concern. Learn more at: http://driveinnovations.org/
Emory-discovered antiviral is poised for COVID-19 clinical trials
The nucleoside inhibitor has advantages over Gilead’s remdesivir but has yet to be tested in humans
Asmall-molecule antiviral discovered by Emory University chemists could soon start human testing against COVID-19, the respiratory disease caused by the novel coronavirus. That’s the plan of Ridgeback Biotherapeutics, which licensed the compound, EIDD-2801, from an Emory nonprofit.
But remdesivir can only be given intravenously, meaning it would be difficult to deploy widely. In contrast, EIDD-2801 can be taken in pill form, says Mark Denison, a coronavirus expert and director of the infectious diseases division at Vanderbilt Medical School. Denison partnered with Emory and researchers at the University of North Carolina to test the compound against coronaviruses.
EIDD-2801 has other promising features. Many antivirals work by introducing errors into the viral genome, but, unlike other viruses, coronaviruses can fix some mistakes. In lab experiments, EIDD-2801 “was able to overcome the coronavirus proofreading function,” Denison says.
He also notes that while remdesivir and EIDD-2801 both block RNA polymerase, they appear to do it in different ways, meaning they could be complementary.
Unlike remdesivir, EIDD-2801 lacks human safety data. Ridgeback founder and CEO Wendy Holman says she expects the US Food and Drug Administration to give the green light for a Phase I study in COVID-19 infections within “weeks, not months.”
“weeks, not months.”
![]() |
|
Clinical data | |
---|---|
Other names | MK-4482, EIDD-2801 |
Legal status | |
Legal status |
|
Identifiers | |
CAS Number | |
PubChem CID | |
UNII | |
Chemical and physical data | |
Formula | C13H19N3O7 |
Molar mass | 329.31 g·mol−1 |
3D model (JSmol) | |
////////EIDD 2801, EMORY, CORONA VIRUS, COVID 19, mk 4482, molnupiravir, merck
CC(C)C(=O)OC[C@H]2O[C@@H](N1C=CC(=NC1=O)NO)[C@H](O)[C@@H]2O
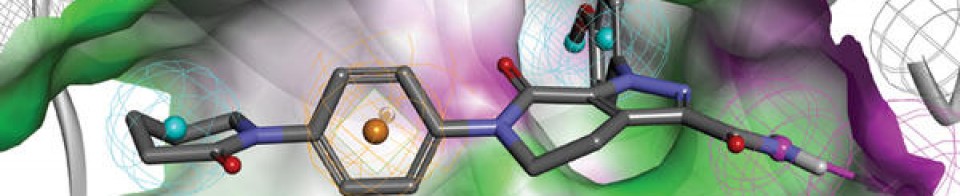
NEW DRUG APPROVALS
ONE TIME
$10.00



Application Id | Application Number | Application Date | Country | Title |
US333828014 | 17170172 | 08.02.2021 | US | N4-HYDROXYCYTIDINE AND DERIVATIVES AND ANTI-VIRAL USES RELATED THERETO |
US305251595 | 16755779 | 07.12.2018 | US | N4-HYDROXYCYTIDINE AND DERIVATIVES AND ANTI-VIRAL USES RELATED THERETO |
WO2021159044 | PCT/US2021/016984 | 07.02.2021 | WO | N4-HYDROXYCYTIDINE AND DERIVATIVES AND ANTI-VIRAL USES RELATED THERETO |
WO2021137913 | PCT/US2020/054857 | 08.10.2020 | WO | 4′-HALOGEN CONTAINING NUCLEOTIDE AND NUCLEOSIDE THERAPEUTIC COMPOSITIONS AND USES RELATED THERETO |


