Home » Uncategorized
Category Archives: Uncategorized
INAXAPLIN
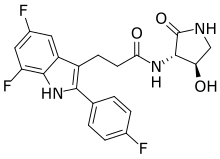
INAXAPLIN, 2446816-88-0
- 2446816-88-0
- S2SJ2RVZ6Y
- UNII-S2SJ2RVZ6Y
- C21H18F3N3O3
3-[5,7-difluoro-2-(4-fluorophenyl)-1H-indol-3-yl]-N-[(3S,4R)-4-hydroxy-2-oxopyrrolidin-3-yl]propanamide
Inaxaplin (VX-147) is a small-molecule apolipoprotein L1 inhibitor developed by Vertex Pharmaceuticals for APOL1-mediated kidney disease. In preliminary studies the drug has shown promise in treating people with kidney disease and multiple gain of function mutations on the APOL1 gene.[1][2]
FSGS is a disease of the podocyte (glomerular visceral epithelial cells) responsible for proteinuria and progressive decline in kidney function. NDKD is a disease characterized by hypertension and progressive decline in kidney function. Human genetics support a causal role for the G1 and G2 APOL1 variants in inducing kidney disease. Individuals with 2 APOL1 risk alleles are at increased risk of developing primary (idiopathic) FSGS, human immunodeficiency virus (HIV)-associated FSGS, and NDKD. Currently, FSGS and NDKD are managed with symptomatic treatment (including blood pressure control using blockers of the renin angiotensin system), and patients with FSGS and heavy proteinuria may be offered high dose steroids. Corticosteroids induce remission in a minority of patients and are associated with numerous side effects. These patients, in particular individuals of recent sub-Saharan African ancestry with 2 APOL1 risk alleles, experience rapid disease progression leading to end-stage renal disease (ESRD). Thus, there is an unmet medical need for treatment for FSGS and NDKD.
PATENT
Compound 2

3-[5,7-difluoro-2-(4-fluorophenyl)-1H-indol-3-yl]-N-[(3S,4R)-4-hydroxy-2-oxo-pyrrolidin-3-yl]propanamide (2)
Synthesis of 3-[5,7-difluoro-2-(4-fluorophenyl)-1H-indol-3-yl]-N-[(3S,4R)-4-hydroxy-2-oxo-pyrrolidin-3-yl]propanamide (2)
Alternative Procedure for Synthesis of Compound (2)
Step 1. Synthesis of Methyl (E)-3-[5,7-difluoro-2-(4-fluorophenyl)-1H-indol-3-yl]prop-2-enoate (C51)
Step 2. Synthesis of Methyl 3-[5,7-difluoro-2-(4-fluorophenyl)-1H-indol-3-yl]propanoate (C52)
Step 3. Synthesis of 3-[5,7-difluoro-2-(4-fluorophenyl)-1H-indol-3-yl]propanoic Acid (S12)
Step 4. Synthesis of 3-[5,7-difluoro-2-(4-fluorophenyl)-1H-indol-3-yl]-N-[(3S,4R)-4-hydroxy-2-oxo-pyrrolidin-3-yl]propanamide (2)
Re-Crystallization of Compound 2
Form A of Compound 2
Hydrate Form A of Compound 2
200 mg of Compound 2 was charged with 10 mL of water. The slurry was cooled to 5° C. and allowed to stir. Hydrate A was observed after 3 days of stirring. |
PATENT
PATENT

AS ON DEC2021 3,491,869 VIEWS ON BLOG WORLDREACH AVAILABLEFOR YOUR ADVERTISEMENT

join me on Linkedin
Anthony Melvin Crasto Ph.D – India | LinkedIn
join me on Researchgate
RESEARCHGATE
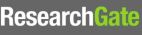
join me on Facebook
Anthony Melvin Crasto Dr. | Facebook
join me on twitter
Anthony Melvin Crasto Dr. | twitter
+919321316780 call whatsaapp
EMAIL. amcrasto@gmail.com
//////////
Clinical data | |
---|---|
Other names | VX-147 |
Legal status | |
Legal status | Investigational |
Identifiers | |
showIUPAC name | |
CAS Number | 2446816-88-0 |
PubChem CID | 147289591 |
ChemSpider | 115009344 |
UNII | S2SJ2RVZ6Y |
ChEMBL | ChEMBL5083170 |
Chemical and physical data | |
Formula | C21H18F3N3O3 |
Molar mass | 417.388 g·mol−1 |
3D model (JSmol) | Interactive image |
showSMILES | |
showInChI |
References
- ^ Gbadegesin, Rasheed; Lane, Brandon (August 2023). “Inaxaplin for the treatment of APOL1-associated kidney disease”. Nature Reviews Nephrology. 19 (8): 479–480. doi:10.1038/s41581-023-00721-0. PMC 10461697. PMID 37106136.
- ^ Egbuna, Ogo; Zimmerman, Brandon; Manos, George; Fortier, Anne; Chirieac, Madalina C.; Dakin, Leslie A.; Friedman, David J.; Bramham, Kate; Campbell, Kirk; Knebelmann, Bertrand; Barisoni, Laura; Falk, Ronald J.; Gipson, Debbie S.; Lipkowitz, Michael S.; Ojo, Akinlolu; Bunnage, Mark E.; Pollak, Martin R.; Altshuler, David; Chertow, Glenn M. (16 March 2023). “Inaxaplin for Proteinuric Kidney Disease in Persons with Two APOL1 Variants”. New England Journal of Medicine. 388 (11): 969–979. doi:10.1056/NEJMoa2202396. PMID 36920755. S2CID 257534251.
………///////INAXAPLIN
Golcadomide (CC-99282)

Golcadomide (CC-99282)
Molecular Weight | 535.57 |
Formula | C28H30FN5O5 |
CAS No. | 2379572-34-4 |
(S)-2-(2,6-Dioxopiperidin-3-yl)-4-((2-fluoro-4-((3-morpholinoazetidin-1-yl)methyl)benzyl)amino)isoindoline-1,3-dione
2-[(3S)-2,6-dioxopiperidin-3-yl]-4-[[2-fluoro-4-[(3-morpholin-4-ylazetidin-1-yl)methyl]phenyl]methylamino]isoindole-1,3-dione
- 2-[(3S)-2,6-Dioxo-3-piperidinyl]-4-[[[2-fluoro-4-[[3-(4-morpholinyl)-1-azetidinyl]methyl]phenyl]methyl]amino]-1H-isoindole-1,3(2H)-dione (ACI)
- (S)-2-(2,6-Dioxopiperidin-3-yl)-4-[[2-fluoro-4-[(3-morpholinoazetidin-1-yl)methyl]benzyl]amino]isoindoline-1,3-dione
- CC 1007548
- CC 99282
- CC-99282
- CC1007548
- Golcadomide
- WHO 12305
Golcadomide HCl, 2639939-36-7
Chemical Name: CC-99282 Hydrochloride; 2-[(3S)-2,6-Dioxopiperidin-3-yl]-4-[[2-fluoro-4-[(3-morpholin-4-ylazetidin-1-yl)methyl]phenyl]methylamino]isoindole-1,3-dione hydrochloride
Novel potent and orally active cereblon (CRBN) E3 ligase modulator
Ref: https://www.sciencedirect.com/science/article/abs/pii/S0268960X22000686
Golcadomide is a modulator of the E3 ubiquitin ligase complex containing cereblon (CRL4-CRBN E3 ubiquitin ligase), with potential immunomodulating and antineoplastic activities. Upon administration, golcadomide specifically binds to cereblon (CRBN), thereby affecting the ubiquitin E3 ligase activity, and targeting certain substrate proteins for ubiquitination. This induces proteasome-mediated degradation of certain transcription factors, some of which are transcriptional repressors in T-cells. This leads to modulation of the immune system, including activation of T-lymphocytes, and downregulation of the activity of other proteins, some of which play key roles in the proliferation of certain cancer cell types. CRBN, the substrate recognition component of the CRL4-CRBN E3 ubiquitin ligase complex, plays a key role in the ubiquitination of certain proteins.

PATENT
US20220324855
Example 1: Synthesis of (S)-2-(2,6-Dioxopiperidin-3-yl)-4-((2-fluoro-4-((3-morpholinoazetidin-1-yl)methyl)benzyl)amino)isoindoline-1,3-dione (Compound 1)
PATENT
WO2022271557
Example 1: Synthesis of (S)-2-(2,6-dioxopiperidin-3-yl)-4-((2-fluoro-4-((3- morpholinoazetidin-1-yl)methyl)benzyl)amino)isoindoline -1,3-dione

[00242] Synthesis of Ethyl 4-nitro-1,3-dioxo-isoindoline-2-carboxylate (Compound 10): To a solution of 4-nitroisoindoline-1,3-dione (Compound 11, 440 g, 2.29 mol) and TEA (262 g, 2.59 mol, 359 mL) in dry DMF (2.2 L) was cooled to 0 °C and ethyl chloroformate (313 g, 2.89 mol, 275 mL) was added dropwise over 5 minutes. The reaction mixture was stirred at 22 °C for 10 hours. The mixture was slowly added to chilled water (10 L) and the resulting suspension stirred for 5 minutes. The suspension was filtered and the filter cake was washed with water (1 L). The solid was dissolved with ethyl acetate (5 L) and the organic phase was washed with aqueous HCl (1 M, 1 L), water (2 L) and brine (2 L). The organic phase was dried over sodium sulfate , filtered and concentrated to give Compound 10 (360 g, 59%) as a white solid. 1 H NMR (400 MHz CDCl 3 ) δ ppm 8.24 (d, J = 7.6 Hz, 1H), 8.19 (d, J = 8.4 Hz, 1H), 8.06-8.02 (m, 1H), 4.49 (q, J = 7.2 Hz, 2H), 1.44 (t, J = 6.8 Hz, 3H).
[00243] Synthesis of tert-Butyl (4S)-5-amino-4-(4-nitro-1,3-dioxo-isoindolin-2-yl)-5-oxo-pentanoate (Compound 6): To a solution of Compound 10 (165 g, 625 mmol) and DIEA (113 g, 874 mmol, 153 mL) in dry THF (1700 mL) was added tert-butyl (4S)-4,5-diamino-5-oxo-pentanoate hydrochloride ( 149 g, 625 mmol) and heated at reflux for 10 hours. The reaction mixture was concentrated under reduced pressure. The resulting residue was diluted with methyl tert-butyl ether (5 L) and stirred at 20 °C for 1 hour. The suspension was filtered and the filter cake was dissolved with DCM (4 L). The organic phase was washed with water (1.5 L x 3), brine (1.5 L) and dried over sodium sulfate . The organic phase was filtered and concentrated under reduced pressure to give a light yellow oil. The oil was diluted with hexane / ethyl acetate (10/1, 2 L) and stirred until a light yellow suspension formed. The suspension was filtered and the filter cake was triturated and concentrated in vacuum to give Compound 6 (175 g, 74%) as a light yellow solid. 1 H NMR (400 MHz CDCl3) δ ppm 8.12 (d, J = 8.0 Hz, 2H), 7.94 (t, J = 8.0 Hz, 1H), 6.48 (s, 1H), 5.99 (s, 1H), 4.84- 4.80 (m, 1H), 2.49-2.44 (m, 2H), 2.32-2.27 (m, 2H), 1.38 (s, 9H).
[00244] Synthesis of tert-Butyl (S)-5-amino-4-(4-amino-1,3-dioxoisoindolin-2-yl)-5-oxopentanoate (Compound 5): To a suspension of Compound 6 (170.0 g, 450.5 mmol, 1.00 eq) in DMA (1.00 L) was added palladium on carbon (50.0 g, 10% purity) under nitrogen. The suspension was degassed under vacuum and purged with hydrogen gas several times. The mixture was stirred under hydrogen gas (50 psi) at 25 °C for 16 hours. The mixture was filtered and the filtrate was poured into cooled water (3.0 L). The mixture was stirred at 10 °C for 1 hour and filtered. The filter cake was washed with water (700 mL) and dissolved in DCM (1.00 L). The organic phase was dried over sodium sulfate , filtered and concentrated under reduced pressure to give Compound 5 (107 g, 68%) as a green solid. 1 H NMR (400 MHz DMSO-d 6 ) δ ppm 7.52 (s, 1H), 7.43 (dd, J = 8.4, 7.2 Hz, 1H), 7.13 (s, 1H), 6.95-6.99 (m, 2H), 6.42 (s, 2H), 5.75 (s, 1H), 4.47-4.51 (m, 1H), 2.32-2.33 (m, 1H), 2.14-2.20 (m, 3H), 1.32 (s, 9H); HPLC purity, 100.0%; SFC purity, 100.0% ee.
[00245] Synthesis of 2-fluoro-4-(hydroxymethyl)benzaldehyde (Compound 8): To a solution of 4-(((tert-butyldimethylsilyl)oxy)methyl)-2-fluorobenzaldehyde (370.0 g, 1.38 mol, 1.00 eq ) in THF (1.85 L) was added a solution of p-toluenesulfonic acid monohydrate (78.7 g, 413.6 mmol, 0.30 eq) in water (1.85 L) drop-wise at 10 °C. The mixture was stirred at 27 °C for 16 hours. TEA (80 mL) was added drop-wise and stirred for 10 minutes. The organic phase was separated and the aqueous phase was extracted with ethyl acetate (600 mL × 4). The combined
organic phase was washed with brine (1.50 L), dried over anhydrous sodium sulfate , filtered and concentrated under reduced pressure. The residue was purified by silica gel column chromatography to give Compound 8 (137.5 g, 76%) as a yellow oil. 1 H NMR (400 MHz CDCl 3 ) δ ppm 10.34 (s, 1H), 7.86 (dd, J = 8.0, 7.2 Hz, 1H), 7.25 (s, 1H), 7.22 (d, J = 4.4 Hz, 1H) , 4.79 (d, J = 6.0 Hz, 2H), 1.91 (t, J = 6.0 Hz, 1H).
[00246] Synthesis of tert-Butyl (S)-5-amino-4-(4-((2-fluoro-4-(hydroxymethyl)benzyl)amino)-1,3-dioxoisoindolin-2-yl)-5- oxopentanoate (Compound 2-b): To a solution of Compound 5 (100.0 g, 287.9 mmol, 1.00 eq) and Compound 8 (57.7 g, 374.3 mmol, 1.30 eq) in dry DCM (1.00 L) was added TFA (164.1 g , 1.44 mol, 5.00 eq) at 0 °C. The reaction mixture was stirred at 28 °C for 2 hours. To the solution was added sodium cyanoborohydride (27.1 g, 431.8 mmol, 1.50 eq) at 0 °C. The mixture was stirred at 28 °C for 30 minutes. The reaction mixture was quenched by addition of MeOH (600 mL) and concentrated under reduced pressure. The residue was purified by silica gel column chromatography to give Compound 2-b (110.0 g, 74.0%) as a yellow solid. 1 H NMR (400 MHz, DMSO-d6) δ ppm 7.56 (s, 1H), 7.50 (dd, J = 8.4, 7.2 Hz, 1H), 7.34 (t, J = 8.0 Hz, 1H), 7.02-7.18 ( m, 4H), 6.94-7.01 (m, 2H), 4.57 (d, J = 6.0 Hz, 2H), 4.47-4.53 (m, 3H), 2.31-2.35 (m, 1H), 2.15-2.22 (m, 3H), 1.31 (s, 9H); HPLC purity, 94.0%; SFC purity, 100.0% ee.
[00247] Synthesis of tert-butyl (S)-5-amino-4-(4-((4-(chloromethyl)-2-fluorobenzyl)amino)-1,3-dioxoisoindolin-2-yl)-5-oxopentanoate (Compound 2-a): To a solution of Compound 2-b (100.0 g, 206.0 mmol, 1.00 eq) in NMP (430.0 mL) was added DIEA (79.9 g, 617.9 mmol, 3.00 eq) and MsCl (47.2 g, 411.9 mmol, 2.00 eq) at 0 °C. The ice bath was removed, and the reaction was stirred at 28°C for 10 hours. The reaction was poured into cooled water (<10°C, 2.0 L) and stirred for 10 minutes. The mixture was extracted with methyl tert-butyl ether (750 mL x 3). The combined organic layer was washed with brine (1.25 L), dried over sodium sulfate , filtered and concentrated under reduced pressure. The residue was purified by silica gel column chromatography to give Compound 2-a (86.0 g, 81.2%) as a yellow solid.
1 H NMR (400 MHz DMSO-d 6 ) δ ppm 7.55 (s, 1H), 7.50 (dd, J = 8.4, 7.2 Hz, 1H), 7.38 (t, J = 8.0Hz, 1H), 7.31 (dd, J = 10.8, 1.6 Hz, 1H), 7.23 (dd, J = 8.0, 1.6 Hz, 1H), 7.16 (s, 1H), 7.11 (t, J = 6.4 Hz, 1H), 7.00 (d, J = 7.2 Hz, 1H), 6.95 (d, J = 8.4 Hz, 1H), 4.74 (s, 2H), 4.61 (d, J = 6.4 Hz, 2H), 4.49-4.53 (m, 1H), 2.29-2.38 (m , 1H), 2.16-2.25 (m, 3H), 1.30 (s, 9H); HPLC purity, 98.0%; SFC purity, 100.0% ee.
[00248] Synthesis of tert-butyl (S)-5-amino-4-(4-((2-fluoro-4-((3-morpholinoazetidin-1-yl)methyl)benzyl)amino)-1,3- dioxoisoindolin-2-yl)-5-oxopentanoate (Compound 2): To a solution of 4-(azetidin-3-yl)morpholine hydrochloride (Compound 7 HCl, 30.5 g, 170.7 mmol, 1.00 eq) and DIEA (66.2 g, 512.0 mmol, 3.00 eq) in DMSO (350.0 mL) was added to a solution of Compound 2-a (86 g, 170.65 mmol, 1.00 eq) in DMSO (350.0 mL) drop-wise at 15 °C. The reaction mixture was stirred at 28 °C for 16 hours. The reaction mixture was poured into cold half saturated brine (<10°C, 2.5 L) and extracted with ethyl acetate (1.50 L, 1.00 L, 800.0 mL). The combined organic phase was washed with saturated brine (1.50 L), dried over sodium sulfate , filtered, and concentrated under reduced pressure. The residue was purified by silica gel column chromatography to give Compound 2 (68.3 g, 65.7%) as a yellow solid. 1 H NMR (400 MHz DMSO-d 6 ) δ ppm 7.55 (s, 1H), 7.50 (dd, J = 8.4, 7.2 Hz, 1H), 7.31 (t, J = 8.0 Hz, 1H), 7.16 (s, 1H), 6.94-7.10 (m, 5H), 4.56 (d, J = 6.4 Hz, 2H), 4.49-4.52 (m, 1H), 3.54-3.55 (m, 6H) 3.31-3.32 (m, 3H), 2.81-2.88 (m, 3H), 2.29-2.38 (m, 1H), 2.15-2.25 (m, 7H), 1.30 (s, 9H); HPLC purity, 100.0%; SFC purity, 100.0% ee.
[00249] Synthesis of (S)-2-(2,6-dioxopiperidin-3-yl)-4-((2-fluoro-4-((3-morpholinoazetidin-1-yl)methyl)benzyl)amino)isoindoline -1,3-dione (Compound 1): A solution of Compound 2 (30.0 g, 49.2 mmol, 1.00 eq) and benzenesulfonic acid (31.1 g, 196.8 mmol, 4.00 eq) in acetonitrile (480.0 mL) was stirred at reflux for 3 hours. The reaction was cooled to 20 °C, poured into cold brine:saturated sodium bicarbonate solution (1:1, <10 °C, 2.0 L) and extracted with ethyl acetate (1.0 L). The organic phase was washed with cold brine:saturated sodium bicarbonate solution (1:1, <10°C, 1.00 L) once more. The combined aqueous phase was extracted with ethyl acetate (500.0 mL x 2). The combined organic phase was washed with cold brine (<10°C, 1.0 L), dried over sodium sulfate , filtered and concentrated under reduced pressure. The crude product was purified by silica gel column chromatography to give Compound 1 (17.5 g, 66.0%) as a yellow solid. 1 H NMR (400 MHz, DMSO-d 6 ) δ ppm 11.10 (s, 1H), 7.54 (t, J = 8.0 Hz, 1H), 7.30 (t, J = 8.0 Hz, 1H), 7.04-7.10 (m , 4H), 7.00 (d, J = 8.4 Hz, 1H), 5.07 (dd, J = 12.8, 5.2 Hz, 1H), 4.58 (d, J = 6.4 Hz, 2H), 3.53-3.55 (m, 6H) , 3.30-3.32 (m, 2H), 2.81-2.89 (m, 4H), 2.54-2.61 (m, 2H), 2.20 (m, 4H) 2.03-2.06 (m, 1H); HPLC purity, 100.0%; SFC purity, 97.2% ee; LCMS (ESI) m/z 536.1 [M+H] + .
Example 2: Synthesis of (S)-2-(2,6-dioxopiperidin-3-yl)-4-((2-fluoro-4-((3- morpholinoazetidin-1-yl)methyl)benzyl)amino)isoindoline -1,3-dione

[00250] Synthesis of tert-butyl (S)-5-amino-4-(4-nitro-1,3-dioxoisoindolin-2-yl)-5-oxopentanoate (Compound 6): Ethyl acetate (245 mL, 5 V ), 3-nitrophthalic anhydride (49.1 g, 0.25 mol, 1 eq), and tert-butyl (S)-4,5-diamino-5-oxopentanoate hydrochloride (59.2 g, 0.25 mol,
1 eq) were charged into a reactor and cooled to 15-20°C. A premade solution of CDI (66.7 g,
0.41 mol, 1.5 eq) in DMF (245 mL, 5 V) was charged and the mixture was stirred at 20-25°C for
1 hour. The reaction was quenched with 15% (wt/wt) aqueous citric acid solution (10 V).
EtOAc (5 V) was added, the mixture was agitated and the phases split and separated. Tea
aqueous layer was extracted with EtOAc (5 V) and the combined organic layers were washed
twice with a 5% (wt/wt) aqueous citric acid solution (5 V each wash). The organic layer was
distilled at reduced pressure to 5 V and further continuously distilled at reduced pressure with the addition of iPrOH (10 V), maintaining a constant volume at 5 V. The final distillate was diluted to 13 V with iPrOH and used in the next step without further handling. 91% solution yield. [00251] Synthesis of tert-butyl (S)-5-amino-4-(4-amino-1,3-dioxoisoindolin-2-yl)-5-oxopentanoate (Compound 5): The solution of Compound 6 in iPrOH was charged to a hydrogenation reactor. 10% palladium on carbon (50% wet, 4.65g 5 wt%) was charged. The reaction mixture was stirred under 50-60 psi H2 at 40-50 o C for 16 hrs. The reaction mixture was filtered and the filter cake was washed three times with iPrOH (1 V each wash). The solution was distilled at reduced pressure to 5 V, cooled to ambient temperature and seeded (1 wt%). Water (20 V) was charged at 20-25 o C. The resulting slurry was cooled to 3-8 o C for 4-8 hrs. The solids were collected by filtration and washed three times with cold water (1.5 V each wash). The solids were dried at 35-45 o C under reduced pressure to give Compound 5 in 87% yield. 1 H NMR (500 MHz DMSO-d 6 ) δ (ppm): 7.52 (s, 1H), 7.43 (dd, J = 8.4, 7.0 Hz, 1H), 7.13 (s, 1H), 6.97 (ddd, J = 10.9, 7.7, 0.61 Hz, 2H), 6.43 (s, 2H), 4.49 (m, 1H), 2.33 (m, 1H), 2.17 (m, 3H), 1.32 (s, 9H); HPLC purity, 99.2%; Chiral purity, 99.9% ee; LCMS (ESI) m/z 348.2, [M+H] + , 292.2 [Mt-Bu+H] + . Residual IPA: 0.7 mol% by 1 H NMR.
[00252] Synthesis of 4-(1-(4-bromo-3-fluorobenzyl)azetidin-3-yl)morpholine (Compound 4): A mixture of 4-bromo-3-flurobenzaldehyde (Compound 14, 82 g, 396 mmol ) and 4-(azetidin-3-yl)-morpholine hydrochloride (Compound 7 HCl, 72 g, 396 mmol) in acetonitrile (820 ml) was agitated at 25±5°C for at least 3 hours. The mixture was cooled to 10±5°C and sodium triacetoxyborohydride (130 g, 594 mmol) was added in four portions while maintaining the temperature of the mixture below 30°C. The temperature of the mixture was adjusted to 25±5°C and stirred for at least 30 min until reaction completion. The mixture was transferred to a precooled (10-15°C) solution of aqueous citric acid (152 g in 400 ml water, 792 mmol) while maintaining the temperature below 30°C. Once the quenching process was complete, the mixture was concentrated to ~ 560 ml (7 volumes) while keeping the temperature at or below 45°C. The mixture was then washed with toluene (320 ml). To the aqueous phase was added THF and the pH was adjusted to above 12 with aqueous NaOH solution (320 ml, 10 N). The phases were separated, and the aqueous phase was removed. The organic phase was washed with brine and subsequently concentrated with addition of THF (~ 3L) until KF ≤ 0.10%. The mixture was filtered to remove any inorganics and the product Compound 4 was isolated as a solution in THF with 95% yield.
[00253] Synthesis of sodium (2-fluoro-4-((3-morpholinoazetidin-1-yl)methyl)phenyl) (hydroxy)methanesulfonate (Compound 13): A solution of Compound 4 (520 g, 1.58 mol) in
THF (380 ml) was cooled to −15 ± 5 °C. A solution of iPrMgCl . LiCl (1.3 M, 1823 ml, 2.37 mol) in THF was added over the course of at least 1 hour while maintaining the temperature below −10 °C. After addition was complete, the temperature of the reaction mixture was adjusted to 0 ± 5 °C and stirred for at least 1 hour. Once magnesiation was complete, the mixture was cooled to −15 ± 5 °C (target −15 °C to −20 °C) and a solution of DMF (245 ml g, 3.16 mol) in THF (260 ml) was added slowly over the course of at least 1 hour while maintaining the temperature below −10 °C. The temperature of the mixture was then adjusted to −15 ± 5 °C and agitated for at least 4 hours.
[00254] Upon reaction completion, the reaction mixture was charged into an aqueous 3 N HCl solution (2600 ml) over the course of at least 1 hour while maintaining the temperature below −5 °C. The temperature of the mixture was then adjusted to 5 ± 5 °C and agitation was stopped, letting the mixture settle for at least 15 minutes. The layers were separated. The lower aqueous layer containing the product was washed with 2-MeTHF (2600 ml). The aqueous layer was then charged with 2-MeTHF (2600 ml) and the temperature of the batch was adjusted to −10 ± 5 °C. To the cooled mixture, an aqueous 5 N NaOH (728 ml, 3.64 mol) solution was added while maintaining the temperature below −5 °C until the pH of the mixture was between 10 and 11. The temperature of the mixture was adjusted to 5 ± 5 °C and agitated for at least 15 minutes. The agitation of the mixture was stopped and the mixture allowed to settle for at least 15 minutes. The layers were separated, and the lower aqueous layer was back extracted two times with 2-MeTHF (2600 ml). The combined organic layer was washed with water (1040 mL) and the organic solution was evaporated to dryness, affording 372 g of crude Compound 3 freebase as an oil (yield 85%). 1 H NMR (DMSO-d 6 ) δ (ppm): 10.18 (s, 1H), 7.78 (t, J =7.7 Hz, 1H), 7.23-7.35 (m, 2H), 3.66 (s, 2H), 3.51 -3.60 (m, 4H), 3.26-3.47 (m, 2H), 2.72-2.97 (m, 3H), 2.12-2.32 (m, 4H).
[00255] The crude Compound 3 freebase (4.3 kg) was adsorbed onto silica gel (8.6 kg) with 100% DCM, loaded onto a 60 L column containing 12.9 kg silica gel (packed with 100% DCM), and eluted with DCM ( 86 L), followed successively by 1% MeOH/DCM (40 L), 3% MeOH/DCM (80 L) and 10% MeOH/DCM (40 L). The fractions were collected and concentrated at or below 38˚C to give Compound 3 as a purified oil (3.345 kg, yield 66%).
[00256] A portion of Compound 3 (1.0 kg, 3.59 mol) was dissolved in ethanol (16.0 L, 16
vol) at 20±5 °C and the mixture heated to 40 °C. A solution of Na2S2O5 (622.0 g, 3.27 mol; 0.91 eq) in water (2 L, 2 vol) was prepared at 20±5 °C and added to the freebase solution at 40 °C to obtain an off-white suspension. The batch was agitated and maintained at 40 °C for 2 hrs, then cooled to 20±5 °C and agitated for 1 to 2 hrs. The batch was filtered and washed with ethanol (2×2.0 L, 2×2 vol) to obtain an off-white solid. The wet cake was dried under vacuum at 40 °C for 18 hrs to afford about 1.88 kg of Compound 13.
[00257] Synthesis of 2-fluoro-4-((3-morpholinoazetidin-1-yl)methyl)benzaldehyde (Compound 3): Compound 13 (1.88 kg) was dissolved in ethyl acetate (15.0 L) at 20±5 °C . A 2 M Na 2 CO 3 solution (total 15.0 L used) was added to adjust the pH to 10.0. The batch was agitated for 1 to 1.5 hrs at 20±5 °C. After the reaction was complete, the phases were separated and the organic layer was washed with brine (2.0 L). The organic layer was concentrated to dryness at 35-38 °C to afford 852.0 g of Compound 3 as a colorless oil (yield 81%).
[00258] Synthesis of 2-fluoro-4-((3-morpholinoazetidin-1-yl)methyl)benzaldehyde bis-oxalic acid salt (Compound 3 bis-oxalic acid salt): A portion of the Compound 3 oil (187 g, 0.67 mol) was dissolved in isopropanol (1125 ml) and water (375 ml). A first portion (~30%) of this freebase mixture (480 ml) was slowly added over the course of at least 30 minutes to a solution of oxalic acid (125 g, 1.38 mol) in IPA (1125 ml)/water (375 ml) at 60 ± 5 °C. A second portion (~20%) of the freebase mixture (320 ml) was slowly added over the course of at least 30 minutes to the reaction mixture at 60 ± 5 °C. The reaction mixture was agitated at 60 ± 5 °C for at least 90 minutes. A third portion (~25%) of the freebase mixture (~ 400 ml) was slowly added over the course of at least 30 minutes to the reaction mixture at 60 ± 5 °C and the reaction mixture was agitated at 60 ± 5 °C for at least 90 minutes. The remaining freebase solution (400 ml) was slowly added over the course of at least 30 minutes to the reaction mixture at 60 ± 5 °C and the reaction mixture was agitated at 60 ± 5 °C for at least 90 minutes. The temperature of the mixture was adjusted to 20 ± 5 °C (target 20 °C) over the course of at least 1 hour and the mixture was agitated for at least 16 hours at 20 ± 5 °C and then filtered. The cake was washed three times with IPA (2 x 375 ml) and dried in the drying oven at ≤ 40 °C with a slow bleed of nitrogen to afford 261 g of Compound 3 bis-oxalic acid salt (yield 85%). 1 H NMR (DMSO-d 6 ) δ (ppm): 10.21 (s, 1H), 7.87 (t, J = 7.6 Hz, 1H), 7.42-7.56 (m, 2H), 4.31 (s, 2H), 3.89 -4.03 (m, 2H), 3.75-3.89 (m, 2H), 3.60 (br t, J = 4.3 Hz, 4H), 3.26 (br t, J = 6.9 Hz, 1H), 2.37 (br s, 4H) .
[00259] Synthesis of tert-butyl (S)-5-amino-4-(4-((2-fluoro-4-((3-morpholinoazetidin-1-yl)methyl)benzyl)amino)-1,3- dioxoisoindolin-2-yl)-5-oxopentanoate (Compound 2):
Acetonitrile (6.8 L, 8.0 X Vol) was added to a 30 L jacketed cylindrical reactor. Compound 5 (0.845 kg, 1.00 X Wt) and Compound 3 bis-oxalic acid salt (1.35 kg, 1.60 The contents of the reactor were equilibrated with agitation at 20 ± 5 °C. Trifluoroacetic acid (0.19 L, 0.22 X Vol) was added dropwise, maintaining the batch temperature at 20 ± 5 °C. The reaction mixture was stirred at 20 ± 5 °C for no less than 5 minutes and then sodium triacetoxyborohydride (0.13 kg, 015 X Wt) was added as a solid, maintaining the batch temperature at 20 ± 5 °C. The process of adding trifluoroacetic acid and then sodium triacetoxyborohydride was repeated an additional 5 times. After the last addition, the reaction mixture was sampled to determine the reaction progress. The reaction was held at 20 ± 5 °C overnight. The reaction mixture was then quenched with water (3.4 L, 4.0 X Vol), maintaining the batch temperature at 20 ± 5 °C. The mixture was then stirred at 20 ± 5 °C for no less than 30 minutes and the resulting slurry filtered through a 3 L sintered glass filter, directing the filtrates to clean containers. The reactor was rinsed with acetonitrile (0.4 L, 0.5 X Vol) and the rinse passed through the contents of the 3 L sintered glass filter, directing the filtrate to the containers containing the main batch. The contents of the containers were concentrated to ~5 X Vol under reduced pressure at a bath temperature of no more than 30 °C. The residue was transferred to a clean reactor, was rinsing with 2-MeTHF (2.5 L, 3.0 X Vol) to complete the transfer. Additional 2-MeTHF (10.1 L, 12.0 X Vol) was added to the reactor, followed by water (3.4 L, 4.0 X Vol). The mixture was agitated for no less than 15 minutes at 20 ± 5 °C, then allowed to settle for no less than 10 minutes at 20 ± 5 °C before transferring the bottom aqueous layer to new containers. An aqueous sodium bicarbonate solution (5.3 L, 6.3 X Vol, 9% wt/wt) was added to the reactor with stirring over 30 minutes, maintaining batch temperature no more than 25 °C. The mixture was agitated for no more than 15 minutes at 20 ± 5 °C, then allowed to settle for no less than 10 minutes at 20 ± 5 °C before the bottom aqueous layer was transferred to new containers. The aqueous sodium bicarbonate wash was repeated an additional 2 times to reach a pH of about 6.6 for the spent aqueous layer. A saturated aqueous solution of NaCl(0.85 L, 1.0 X Vol) was then added to reactor with agitation. The mixture was agitated for no less than 15 minutes at 20 ± 5 °C, then allowed to settle for no less than 10 minutes before the bottom aqueous layer was transferred to new containers. The remaining organics were concentrated under reduced pressure to a batch volume of ~5 X Vol at a bath temperature of about 40 °C. Acetonitrile (5.1 L, 6.0 X Vol) was added to the residual volume and the resulting solution concentrated to a batch volume of ~ 5 X Vol under reduced pressure at bath temperature of about 40 °C. The process of adding acetonitrile and concentrating under vacuum was repeated two more times to reach the distillation endpoint with a water content of about 1%. The acetonitrile solution was transferred to a clean container along with two 1.7 L (2.0 X Vol) rinses and held at 5 °C overnight. The acetonitrile solution was then filtered through a 3 L sintered glass filter, followed by a 1.7 L (2.0 X Vol) acetonitrile rinse, directing the filtrates to a clean container. The filtrate was transferred to a clean reactor and the container rinsed twice with 1.7 L (2.0 X Vol) of acetonitrile to complete the transfer. Enough acetonitrile (roughly 0.6 L) was added to adjust the total volume in the reactor to about 14 L. A solution assay of the contents of the reactor was obtained to calculate the amount of Compound 2 present for use in the next step (result = 1.3 kg = 1.00 X Wt for remainder of process).
[00260] Synthesis of (S)-2-(2,6-dioxopiperidin-3-yl)-4-((2-fluoro-4-((3-morpholinoazetidin-1-yl)methyl)benzyl)amino)isoindoline -1,3-dione bis-besylate salt (Compound 1 bis-besylate salt): The solution of Compound 2 in acetonitrile from the previous step was diluted with acetonitrile (roughly 2 L) such that the total volume in the reactor was about 16 L. The solution was cooled with stirring to 10 ± 5 °C and held within that range for 96 hours. Benzenesulfonic acid (1.86 kg, 1.43 X Wt) was added while sparging the reaction mixture with nitrogen gas and maintaining the batch temperature at 10 ± 10 °C. The temperature of the reactor was then adjusted to 20 ± 5 °C and the mixture stirred at that temperature for 60 minutes. The total volume of reaction mixture was adjusted back to 16 L to account for solvent lost during sparging by the addition of acetonitrile (roughly 0.4 L). The reaction mixture was then heated to 55 ± 5 °C over the course of about 30 minutes and held in that range for 15 to 16 hours for reaction completion. The mixture was then cooled to 50 ± 5 °C and MTBE (3.9 L, 3.0 X Vol) was added, maintaining the batch temperature at 50 ± 5 °C. The mixture was allowed to stir at 50 ± 5 °C for about 1.5 hours to establish a self-seeded slurry. Additional MTBE (3.9 L, 3.0 X Vol) was added to the reactor over the course of about 1.75 hours at 50 ± 5 °C. The slurry was cooled to 20 ± 5 °C over the course of about 1.75 hours and held in that temperature range overnight. The slurry was filtered using a Buchner funnel. The reactor was rinsed twice with
MTBE (3.9 L each, 3.0 X Vol) and the rinse was used to wash the solids in the Buchner funnel. The solids were dried on drying trays for about 23 hours at 40 °C under reduced pressure (15-150 mbar), yielding 1.62 kg (77.9%) of Compound 1 bis-besylate salt.
[00261] Synthesis of (S)-2-(2,6-dioxopiperidin-3-yl)-4-((2-fluoro-4-((3-morpholinoazetidin-1-yl)methyl)benzyl)amino)isoindoline -1,3-dione hydrochloride salt (Compound 1 HCl): A suspension of Compound 1 bis-besylate salt (120 g, 1 equiv.) in 2-MeTHF (25 L/kg) was added to a reactor and agitated at 10 °C. A solution of KHCO 3 (32.5 g, 2.4 equiv) in water (1.8 L, 6 L/kg) was added to the slurry over the course of 40 minutes. The mixture was stirred for an additional 30 minutes. The batch was then allowed to settle, at which point the aqueous (bottom) layer was separated and discarded. An aqueous solution of NaCl (5%, 5 L/kg, 575 ml) was added to the organic layer and the mixture was agitated for 10 minutes, after which point the temperature was raised to 20 °C. The batch was allowed to settle, at which point the aqueous (bottom) layer was discarded. The brine was repeated a second time.
Additional 2-MeTHF (500 ml) was added to dilute the organic layer, resulting in a concentration of about 20 mg product per ml. A solution of HCl (total 0.98 eq.) in 2-MeTHF was prepared and a portion (20% of total, corresponding to ~0.2 eq.) then added to the reaction mixture over the course of about 10 min. Seeds of Compound 1 hydrochloride (~5% wt) were added, but did not dissolve. The batch was held under vigorous agitation for one hour. To the slurry, the remaining portion of the HCl solution (~0.78 eq.) was added over the course of 3 hours at a constant rate. Vigorous agitation was maintained. After addition was complete, the batch was held for one hour, after which the batch was filtered, washed three times with 3 L/kg of 2-MeTHF . The filter cake was placed in a vacuum oven at 22 °C for 12 hours, at which point the temperature was raised to 40 °C. Dry cake of Compound 1 hydrochloride (58g, 75% yield) was obtained and packaged. Achiral HPLC purity: 98.91%; chiral HPLC purity: 99.68%.
Example 4: Synthesis of (S)-2-(2,6-dioxopiperidin-3-yl)-4-((2-fluoro-4-((3- morpholinoazetidin-1-yl)methyl)benzyl)amino)isoindoline -1,3-dione

[00264] Synthesis of tert-butyl (S)-5-amino-4-(4-nitro-1,3-dioxoisoindolin-2-yl)-5-oxopentanoate (Compound 6): To a solution of 3-nitrophthalic anhydride (Compound 12,
35.15 g, 176.6 mmol, 1.00 eq) in ethyl acetate (350 mL) was added tert-butyl (4S)-4,5-diamino-5-oxo-pentanoate hydrochloride (Compound 9 HCl, 43.22 g, 181.1 mmol, 1.025 eq) ), DMF (70
mL) and 2-MeTHF (110 mL) at 25°C. 2,6-Lutidine (23.4 mL, 201 mmol, 1.14 eq) was added
slowly to maintain the temperature at or below 25°C. The mixture was aged at 25°C for 1 hour before being cooled to 5°C. CDI (4.17 g, 25.7 mmol, 0.146 eq) was added and stirred until the temperature returned to 5°C. Another portion of CDI (4.62 g, 28.5 mmol, 0.161 eq) was added and stirred until temperature returned to 5°C. CDI (8.87 g, 54.7 mmol, 0.310 eq) was added and stirred until the temperature returned to 5°C. CDI (8.91 g, 54.9 mmol, 0.311 eq) was added and stirred until the temperature returned to 5°C. The mixture was warmed to 20°C and CDI (16.4 g, 101.1 mmol, 0.573 eq) was added, and the mixture was aged at 20°C for 16 hours. The mixture was cooled to 5°C and a solution of 30 wt% citric acid and 5 wt% NaCl (350 mL) was added slowly while maintaining the temperature. The mixture was warmed to 20°C and aged for 30 minutes. The phases were split and separated. The organic phase was diluted with EtOAc (175 mL) and washed with a solution of 5 wt% citric acid (175 mL), and concentrated by distillation (75 torr, 50°C) to a volume of 175 mL EtOAc. The solvent was changed to iPrOH by constant volume distillation (75 torr, 50°C) with 350 mL iPrOH to a final volume of 175 mL. The distillate was diluted with 200 mL iPrOH to afford Compound 6 as a solution for use in the next step. 1 H NMR (500 MHz, CDCl 3 ) δ (ppm): 8.18 – 8.13 (m, 2H), 7.96 (t, J = 7.8 Hz, 1H), 6.34 (s, 1H), 5.59 (s, 1H), 4.90 (dd, J = 10.1, 4.6 Hz, 1H), 2.61 (ddt, J = 14.6, 10.1, 6.1 Hz, 1H), 2.49 (ddt, J = 14.2, 8.7, 5.2 Hz, 1H), 2.44 – 2.29 ( m, 2H), 1.44 (s, 9H).
[00265] Synthesis of tert-butyl (S)-5-amino-4-(4-amino-1,3-dioxoisoindolin-2-yl)-5-oxopentanoate (Compound 5): To a solution of Compound 6 in iPrOH (375 mL) was added 5% palladium on carbon (1.23 g, 3.5 wt%, wet). The mixture was purged with nitrogen five times and with hydrogen three times. The mixture was pressurized with hydrogen (50 psi) and aged at 50°C for 16 hours. The mixture was cooled to room temperature and purged with nitrogen three times, filtered to remove catalyst, and the filter cake was washed with iPrOH (20mL) three times. The filtrate was concentrated to 200 mL, seeded (0.454 g, 1.3 wt%) at 22 °C, and aged for 45 minutes. Water (1325 mL) was added over 3 hours at 22°C. After the addition of water, the mixture was cooled to 8°C over 2 hours and aged for 1 hour at 8°C. The slurry was filtered, and the cake was rinsed with cold water (200 mL) three times and dried under vacuum at 50°C to yield Compound 5 as a yellow solid (47.97 g, 80.6% yield, 99.62% LC purity, 103% 1 H NMR potency). 1 H NMR (500 MHz, CDCl 3 ) δ (ppm): 7.46 (dd, J = 8.3, 7.0 Hz, 1H), 7.19 (d, J = 7.2 Hz, 1H), 6.89 (d, J = 8.3 Hz, 1H), 6.28 (s, 1H), 5.41 (s, 1H), 5.28 (s, 2H), 4.83 (dd, J = 9.3, 6.0 Hz, 1H), 2.52 (p, J = 7.0 Hz, 2H), 2.36 – 2.29 (m, 2H), 1.44 (s, 9H). 13 C NMR (126 MHz, CDCl 3 ) δ (ppm): 171.80, 171.12, 169.64, 168.27, 145.70, 135.50, 132.20, 121.43, 112.98, 80.99, 53.04, 32.23, 28.02 , 24.36. LCMS (ESI): m/z 291.9 [M+H – tBu]
[00266] Synthesis of 4-(1-(4-bromo-3-fluorobenzyl)azetidin-3-yl)morpholine bis-methanesulfonic acid salt (Compound 4 bis-methanesulfonic acid salt): A mixture of 4-bromo-3- fluorobenzaldehyde (Compound 14, 102 g, 493 mmol) and 4-(azetidin-3-yl)morpholine hydrochloride (Compound 7 HCl, 90 g, 493 mmol) in acetonitrile (1000 ml) was agitated at a temperature of about 20 to 25 °C for 2 to 3 hours. The slurry was cooled to temperature of about 10 to 15 °C and sodium triacetoxyborohydride (STAB, 162 g, 739 mmol) was added in 4 portions over the course of about 45 minutes while maintaining the batch temperature at no more than 30 °C. The slurry was stirred at a temperature of about 20 to 25 °C for at least 30 minutes and then quenched by an aqueous citric acid solution (191 g, 986 mmol in 500 ml of water) at a temperature of about 40 to 45 °C over the course of 2 hours. Upon completion of the quenching process, the batch volume was reduced by vacuum distillation to about 700 ml at a temperature of no more than 45 °C. Cyclopentylmethylether (CPME, 400 ml) was added to the aqueous solution to afford a final volume of about 1100 ml. The pH was adjusted to about 8 to 9 by addition of an aqueous solution of 10 N NaOH (added volume about 430 ml). The phases were separated, and the aqueous phase discarded. The organic phase was washed with brine (100 ml) twice such that the pH was no more than 8 and the volume was adjusted to about 1000 ml with addition of extra CPME. The batch was distilled at constant volume under reduced pressure with addition of CPME until KF was no more than 0.15%. CPME was added (if needed) to adjust the batch to a volume of 1000 ml at the end of distillation. The dry CPME solution was seeded (500 to 750 mg) at ambient temperature. The seeded, dry CPME slurry was heated to a temperature of 50 to 60 °C and then charged with methanesulfonic acid in 200 ml of CPME over the course of 4 to 5 hours. The slurry was then cooled to 20 °C over the course of 4 to 5 hours and kept at 20 °C for 3 to 4 hours, filtered, rinsed with CPME and dried in a vacuum oven at 35 to 40 °C over 16 hours to give Compound 4 bis-methanesulfonic acid salt as a white solid. 1 H NMR (500 MHz DMSO-d 6 ) δ (ppm): 10.62 (br s, 1-2H), 7.85 (t, J = 7.8 Hz, 1H), 7.58 (dd, J = 9.5 Hz, 1.9 Hz, 1H), 7.34 (dd, J = 8.2 Hz, 1.8 Hz, 1H), 4.55-4.24 (m, 7H), 3.84 (br s, 4H), 3.14 (m, 4H); HPLC purity, 99.8%, LCMS (ESI) m/z 329.1 /331.1[M/M+2] + . XRPD pattern of the product is shown in FIG.10. DSC thermogram of the product is shown in FIG.11.
[00267] Preparation of 4-(1-(4-bromo-3-fluorobenzyl)azetidin-3-yl)morpholine (Compound 4): A slurry of Compound 4 bis-methanesulfonic acid salt (70 g, 134 mmol) in t -butyl methyl ether was cooled to 10±5°C. An aqueous solution of NaOH (2 N, 201 ml, 403 mmol) was added over the course of at least 30 minutes while maintaining the batch temperature at about 15 °C. After the addition of NaOH , the batch temperature was raised to 20±5°C and agitated over the course of about 20 minutes. The organic layer was separated and washed with water (210 ml) three times. The organic layer was subsequently concentrated with addition of THF (~ 1.05L) until KF ≤ 0.10%. The product Compound 4 was isolated as a solution in THF
with 95% solution yield.
[00268] Preparation of 2-fluoro-4-((3-morpholinoazetidin-1-yl)methyl)benzaldehyde dihydrochloride (Compound 3 di-HCl): A solution of Compound 4 (44 g, 134 mmol) in THF (total volume ~ 350 ml) was then cooled to −20 ± 5 °C. A solution of iPrMgCl . LiCl (1.3 M, 176 ml, 228 mmol) in THF was added over the course of half an hour while maintaining the temperature below −10 °C. After the addition was complete, the batch was stirred at −20 ± 5 °C for 16 to 22 hours. DMF (21 ml, 268 mmol) was then added slowly over the course of 30 minutes while maintaining the batch temperature no more than -15 °C. The batch was stirred at −20 ± 5 °C for 6 to 24 hours. 2-MeTHF (350 ml) was then added to the batch over the course of 30 minutes, followed by slow addition of 3 N HCl (235 ml, 704 mmol) while keeping the batch temperature no more than -10 °C. After the addition of aqueous HCl, the batch was warmed to 0 ± 5 °C and 2 N aqueous NaOH (154 ml, 309 mmol) was added slowly to adjust the solution pH to about 8 to 9. The batch was stirred for about 30 minutes and then warmed to 20 ± 5 °C. The organic layer was separated and washed with 15% aqueous NaCl (3 x 140 ml). The organic layer was subsequently concentrated with addition of 2-MeTHF until KF ≤ 0.10%.
[00269] A portion of the free base of 2-fluoro-4-((3-morpholinoazetidin-1-yl)methyl)benzaldehyde (37.4 g, 134 mmol) so obtained was dissolved in 2-MeTHF (total ~ 420 ml) , to which isopropanol (420 ml) and water (21 ml) were added at 20 ± 5 °C. The batch was then heated to 50 ± 5 °C and a solution of HCl in IPA (5 to 6 N, 28 ml, half of total HCl volume) was added over the course of 1 hour. The batch was seeded with 2-fluoro-4-((3-morpholinoazetidin-1-yl)methyl)benzaldehyde dihydrochloride (700 mg) and aged for 1 hour. The remaining HCl (28 ml) was then added over the course of 1 hour. The batch was agitated at 50 ± 5 °C for 4 hours and then cooled to 20 ± 5 °C for 8 hours. The slurry was filtered, washed with IPA (210 ml), and the filter cake dried under vacuum at 50 ± 5 °C to afford Compound 3 dihydrochloride salt (36 g, yield 75%). 1 H NMR (DMSO-d 6 ) δ (ppm): 12.32-12.55 (m, 1H), 10.23 (s, 1H), 7.93 (t, J =7.6 Hz, 1H), 7.66 (d, J = 10.5 Hz , 1H), 7.58 (d, J = 7.9 Hz, 1H), 4.80 (br s, 2H), 4.48-4.70 (m, 2H), 4.30 (br s, 4H), 3.78-4.00 (m, 5H), 2.93-3.15 (m, 2H). Two polymorphic forms were obtained. XRPD pattern and DSC thermogram of Form A (anhydrous) are shown in FIG.5 and FIG.6, respectively. XRPD pattern, TGA thermogram and DSC thermogram of Form B (hydrate) are shown in FIG.7, FIG.8, and FIG.9, respectively.
[00270] Synthesis of tert-butyl (S)-5-amino-4-(4-((2-fluoro-4-((3-morpholinoazetidin-1-yl)methyl)benzyl)amino)-1,3- dioxoisoindolin-2-yl)-5-oxopentanoate (Compound 2): A mixture of Compound 5 (12 g, 34.5 mmol, 1.0 eq) and Compound 3 dihydrochloride (14.56 g, 41.5 mmol, 1.2 eq) in MeCN (96 ml) was cooled to 0-5 °C. Trifluoroacetic acid (TFA, 2.0 ml, 26 mmol, 0.75 eq) was added, followed by sodium triacetoxyborohydride (STAB, 2.75 g, 12.95 mmol, 0.375 eq) while maintaining the internal temperature below 10 °C. The addition of TFA and STAB was repeated three additional times. After a total of four additions of TFA and STAB, the reaction was aged at 0-5°C for 1 hour. A 10% brine solution (108 ml) was then added to the reaction mixture over the course of 1 hour and partitioned with IPAc (96 ml). The mixture was warmed to 20-25 °C and aged for 30 minutes. The layers were then separated and the organic layer was washed with 2.0 M K3PO4 (114 ml). The pH of the spent aqueous layer should have a pH of about 8.5 – 9.0. The layers were separated again and the organic phase was washed with 8.5% NaHCO 3 (2 x 60 ml), with 30 minutes between each wash, followed by 24% brine (60 ml). The organic fraction was distilled to 72 ml at an internal temperature near 50°C. Toluene (72 ml) was added to bring the volume to 144 ml and distillation continued at constant volume at 50°C with feed and bleed until water content < 0.1. The mixture was heated to 50°C and acetonitrile (48 ml) was added, followed by slow addition of heptane (144 ml) while maintaining the internal temperature above 45 °C. The reaction was held at 50 °C for 2 hours. Once complete, the reaction was slowly cooled to 20-25°C over the course of 4 hours and held at 20-25°C overnight (16 hour). The yellow slurry was then filtered and the yellow cake displacement washed with 1:3:3 mixture of acetonitrile/heptane/toluene (3 x 48 ml). The final cake was then dried under reduced pressure at 50 °C under nitrogen to provide Compound 2 (87.7% isolated molar yield) with >99.0% LCAP. HPLC purity, 99.85%; Chiral purity, >99.9% ee. 1 H NMR (DMSO-d6, 500 MHz) δ (ppm) 7.55 (s, 1H), 7.51 (dd, J = 7.2, 8.4 Hz, 1H), 7.32 (t, J = 7.9 Hz, 1H), 7.16 ( s, 1H), 7.0-7.1 (m, 5H), 4.57 (d, J = 6.3 Hz, 2H), 4.5-4.5 (m, 1H), 3.5-3.6 (m, 6H), 3.3-3.4 (m, 3H), 2.8-2.9 (m, 3H), 2.3-2.4 (m, 1H), 2.1-2.3 (m, 7H), 1.31 (s, 9H); LCMS m/z 610.3 [M+H] + .
[00271] Synthesis of (S)-2-(2,6-dioxopiperidin-3-yl)-4-((2-fluoro-4-((3-morpholinoazetidin-1-yl)methyl)benzyl)amino)isoindoline -1,3-dione bis-besylate salt (Compound 1 bis-besylate): To a suspension of Compound 2 (130 g, 1.0 equiv.) in MeCN (1.56 L, 12 L/kg) agitated at 55 °C was added a solution of benzene sulfonic acid (185 g, 5.5
equiv.) in MeCN (0.39 L, 3 L/kg) and water (0.01 L, 2.0 equiv.). The mixture was stirred at 55 °C for 16 hours. After the reaction age, crystalline seeds (1.3 g, 1 wt%) of bis-besylate salt of Compound 1 were charged into the batch, resulting in formation of a yellow slurry. The slurry was then cooled to 20 °C over the course of 90 minutes. 2-MeTHF (1.3 L, 10 L/kg) was added to the batch slowly over 2 hours at 20 °C. The batch was agitated for an additional 4 hours at 20°C. The yellow slurry was then filtered and the yellow cake re-slurried with MeTHF (1.3 L, 10 L/kg) followed by a displacement MeTHF (0.65 L, 5 L/kg) wash. The final cake was then dried under reduced pressure at 50 °C under nitrogen to give Compound 1 bis-besylate salt (160 g, 88.4% yield). HPLC purity: 98.39%; chiral HPLC purity: 100%. XRPD pattern, TGA thermogram and DSC thermogram of the product are shown in FIG.1, FIG.2, and FIG.3, respectively.
[00272] Synthesis of (S)-2-(2,6-dioxopiperidin-3-yl)-4-((2-fluoro-4-((3-morpholinoazetidin-1-yl)methyl)benzyl)amino)isoindoline -1,3-dione hydrochloride salt (Compound 1 HCl): A suspension of Compound 1 bis-besylate salt (300 g, 1 equiv.) in EtOAc (4.68 L, 15.6 L/kg) and 2-propanol (0.12 L, 0.4 L/kg) was agitated at 15 °C. To the suspension was added a solution of KHCO 3 (82.4 g, 2.5 equiv) in water (1.8 L, 6 L/kg) over 30 minutes. The mixture was heated to 20 °C over 30-60 minutes and then agitated for 30 minutes. The batch was allowed to settle for 30 minutes, at which point the aqueous (bottom) layer was discarded. To the rich organic layer was added water (1.2 L, 4 L/kg) and the reactor contents were agitated for 30 minutes. The batch was allowed to settle for 30 minutes, at which point the aqueous (bottom) layer was discarded. To the rich organic stream was added 2-propanol (2.375 L, 7.9 L/kg) and the stream was then filtered. Water was added to the filtrate to adjust the water content to 8≤KF≤8.2. To the above agitated solution at 20 °C was added 0.2 N HCl (38 mL, 0.025 equiv prepared in EtOAC/IPA 2:1, v/v with 8wt% water) over 10 minutes. To the mixture was added crystalline seeds of Compound 1 hydrochloride salt (1.6 g, 0.5wt%) and the contents of the reactor were agitated at 20 °C for 30 minutes. To the suspension was added 0.2 N HCl (1.44 L, 0.945 equiv. prepared in EtOAC/IPA 2:1, v/v with 8 wt% water) over 4.5 hours. The slurry was agitated for 14 hours, then filtered and washed with EtOAC/IPA (750 mL, 2.5 L/kg, 2:1 v/v with 8 wt% water) followed by IPA (750 mL, 2.5 L/kg). The solids were dried under vacuum at 40 °C to afford Compound 1 hydrochloride salt (170 g, 90% yield). Achiral HPLC purity: 99.91%; chiral HPLC purity: 99.58%. XRPD analysis (FIG.4) confirmed the product (a)


AS ON DEC2021 3,491,869 VIEWS ON BLOG WORLDREACH AVAILABLEFOR YOUR ADVERTISEMENT

join me on Linkedin
Anthony Melvin Crasto Ph.D – India | LinkedIn
join me on Researchgate
RESEARCHGATE
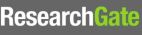
join me on Facebook
Anthony Melvin Crasto Dr. | Facebook
join me on twitter
Anthony Melvin Crasto Dr. | twitter
+919321316780 call whatsaapp
EMAIL. amcrasto@gmail.com
//////////Golcadomide, CC 99282, CC 1007548, WHO 12305
C1CC(=O)NC(=O)C1N2C(=O)C3=C(C2=O)C(=CC=C3)NCC4=C(C=C(C=C4)CN5CC(C5)N6CCOCC6)F
Igermetostat
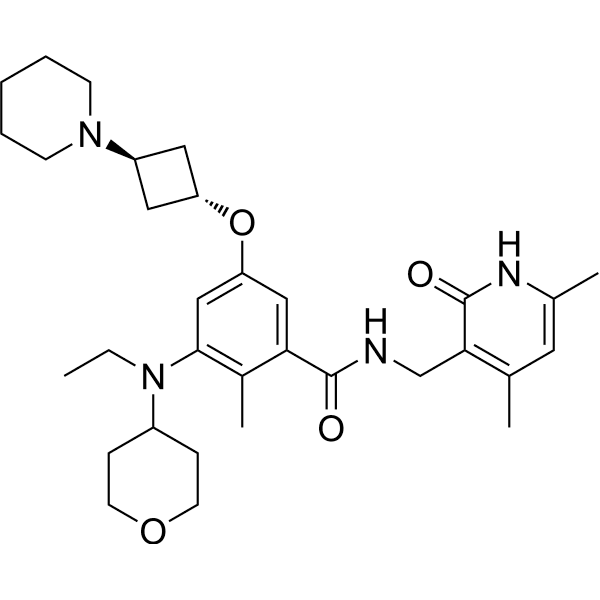
Igermetostat
C32H46N4O4. 550.73
2409538-60-7
2KES6YSH8D
INN 12655
BENZAMIDE, N-((1,2-DIHYDRO-4,6-DIMETHYL-2-OXO-3-PYRIDINYL)METHYL)-3-(ETHYL(TETRAHYDRO-2H-PYRAN-4-YL)AMINO)-2-METHYL-5-((TRANS-3-(1-PIPERIDINYL)CYCLOBUTYL)OXY)-
IGERMETOSTAT
IGERMETOSTAT [INN]
N-((1,2-DIHYDRO-4,6-DIMETHYL-2-OXO-3-PYRIDINYL)METHYL)-3-(ETHYL(TETRAHYDRO-2H-PYRAN-4-YL)AMINO)-2-METHYL-5-((TRANS-3-(1-PIPERIDINYL)CYCLOBUTYL)OXY)BENZAMIDE
N-((4,6-DIMETHYL-2-OXO-1,2-DIHYDROPYRIDIN-3-YL)METHYL)-3-(ETHYL(OXAN-4-YL)AMINO)-2-METHYL-5-(((1R,3R)-3-(PIPERIDIN-1-YL)CYCLOBUTYL)OXY)BENZAMIDE
PATENT
PATENT
US20230382898
The compound has been disclosed in patent WO2020020374A1. It is an enhancer of Zeste homolog 2 (EZH2) inhibitor, and can be used for preventing or treating EZH2-mediated diseases, including brain cancer, thyroid cancer, cardiac sarcoma, lung cancer, oral cancer, stomach cancer and various other cancers.
PATENT
US20210308141
Example 4: Preparation of the Compound N-((4,6-dimethyl-2-oxo-1,2-dihydropyridin-3-yl)methyl)-3-(ethyl(tetrahydro-2H-pyran-4-yl)amino)-2-methyl-5-(trans-3-morpholinylcyclobutoxy)benzamide (4)
The title compound was prepared by referring to Example 1 with cyclobutanol replaced by cis-3-morpholinylcyclobutyl-1-ol. The cis-3-morpholinylcyclobutyl-1-ol was prepared as follows: |
[1]. Peng, et al. Polysubstituted benzene compound and preparation method and use thereof.
World Intellectual Property Organization, WO2020020374 A1. 2020-01-30.
//////////Igermetostat
CCN(C1CCOCC1)c2cc(O[C@H]3C[C@@H](C3)N4CCCCC4)cc(C(=O)NCc5c(C)cc(C)[nH]c5=O)c2C
Oditrasertib,

Obeldesivir,
2647441-36-7
361.35 g/mol
C16H19N5O5
[(2R,3S,4R,5R)-5-(4-aminopyrrolo[2,1-f][1,2,4]triazin-7-yl)-5-cyano-3,4-dihydroxyoxolan-2-yl]methyl 2-methylpropanoate
Q55KCM7PXB; ATV006; UNII-Q55KCM7PXB
Obeldesivir (GS-5245, ATV006) is an isobutyric ester prodrug of GS-441524 made by Gilead Sciences that is currently in Phase III trials for the outpatient treatment of COVID-19 in high risk patients.[1][2] The purpose of the isobutyric ester modification on obeldesivir is to improve the oral bioavailability of the parent nucleoside, GS-441524. Obeldesivir is hydrolyzed to its parent nucleoside, GS-441524, which is in turn converted to remdesivir-triphosphate (GS-443902) by a nucleoside kinase, adenylate kinase and nucleotide diphosphate kinase.[3][4]
GS-443902 is a bioactive ATP analogue with broad-spectrum antiviral activity [5] and is the same compound formed by remdesivir, though by a different enzymatic pathway. Unlike remdesivir, which is metabolized by enzymes that are highly expressed in the liver, GS-441524 released by obeldesivir is metabolized by enzymes that are evenly expressed throughout the body. Due to their different metabolic pathways, obeldesivir can be administered orally, whereas remdesivir must be administered intravenously for COVID-19 treatment.[citation needed]
The pharmacokinetic properties of obeldesivir and improved was first published by Chinese researchers in May 2022. The Chinese group pursued investigation of obeldesivir independently from Gilead Sciences. Compared to IV administered GS-441524 in rats at 5 mg/kg, orally administered obeldesivir at 25 mg/kg (referred to as “ATV006”) yielded approximately 22% bioavailability.[6] Treatment with obdeldesivir reduced viral load and prevents lung pathology in KI-hACE2 and Ad5-hACE2 mouse models of SARS-CoV-2. A patent filed by Gilead Sciences with a priority date of August 27, 2020,[7] found the bioavailability of GS-441524 after oral administration of obdeldesivir (compound 15) in mice, rats, ferrets, dogs, and cynomolgus macaques to be 41%, 63.9%, 154%, 94%, and 38%, respectively. Across all species evaluated, obeldesivir showed improved oral bioavailability compared to oral administration of the parent nucleoside, GS-441524
PAPER
https://pubs.acs.org/doi/10.1021/acs.jmedchem.3c00750
Abstract

Remdesivir 1 is an phosphoramidate prodrug that releases the monophosphate of nucleoside GS-441524 (2) into lung cells, thereby forming the bioactive triphosphate 2-NTP. 2-NTP, an analog of ATP, inhibits the SARS-CoV-2 RNA-dependent RNA polymerase replication and transcription of viral RNA. Strong clinical results for 1 have prompted interest in oral approaches to generate 2-NTP. Here, we describe the discovery of a 5′-isobutyryl ester prodrug of 2 (GS-5245, Obeldesivir, 3) that has low cellular cytotoxicity and 3–7-fold improved oral delivery of 2 in monkeys. Prodrug 3 is cleaved presystemically to provide high systemic exposures of 2 that overcome its less efficient metabolism to 2-NTP, leading to strong SARS-CoV-2 antiviral efficacy in an African green monkey infection model. Exposure-based SARS-CoV-2 efficacy relationships resulted in an estimated clinical dose of 350–400 mg twice daily. Importantly, all SARS-CoV-2 variants remain susceptible to 2, which supports development of 3 as a promising COVID-19 treatment.
Synthesis of ((2R,3S,4R,5R)-5-(4-Aminopyrrolo[2,1-f][1,2,4]triazin-7-yl)-5-cyano-3,4-dihydroxytetrahydrofuran-2-yl)methyl Isobutyrate (3)
To a solution of (3aR,4R,6R,6aR)-4-(4-aminopyrrolo[2,1-f][1,2,4]triazin-7-yl)-6-(hydroxymethyl)-2,2-dimethyltetrahydrofuro[3,4-d][1,3]dioxole-4-carbonitrile 4 (2000 mg, 6.0 mmol) (30) and isobutyric acid (638 mg, 7.2 mmol) in DMF (5 mL), N,N′-diisopropylcarbodiimide (914 mg, 7.2 mmol) was slowly added followed by 4-(dimethylamino)pyridine (737 mg, 6.0 mmol). The reaction mixture was stirred for 4 h and then diluted with ethyl acetate, washed with water and brine, dried over sodium sulfate, and concentrated under reduced pressure. The residue was subjected to silica gel chromatography, eluting with 20% MeOH in CH2Cl2 to provide the intermediate ((3aR,4R,6R,6aR)-6-(4-aminopyrrolo[2,1-f][1,2,4]triazin-7-yl)-6-cyano-2,2-dimethyltetrahydrofuro[3,4-d][1,3]dioxol-4-yl)methyl isobutyrate. LCMS m/z = 402.2 (M + 1). To a solution of the intermediate (1500 mg) in THF (10 mL), conc. HCl (2 mL) was added, and the mixture was stirred at rt for 4 h. The reaction mixture was diluted with CH2Cl2, washed with water, saturated aqueous bicarbonate, and brine, dried over sodium sulfate, concentrated, and subjected to silica gel chromatography, eluting with 30% MeOH in CH2Cl2 to afford the title compound (660 mg, 50%). 1H NMR (400 MHz, MeOH-d4) δ 7.88 (s, 1H), 6.96–6.85 (m, 2H), 4.50–4.27 (m, 4H), 4.16 (dd, J = 6.2, 5.3 Hz, 1H), 2.56 (p, J = 7.0 Hz, 1H), 1.14 (dd, J = 7.0, 3.8 Hz, 6H). LCMS m/z: 362.1 (M + 1). 13C NMR (400 MHz, CHCl3–d3) δ 175.9, 155.6, 147.9, 123.5, 110.2, 100.8, 116.9, 116.6, 81.3, 79.0, 74.0, 70.2, 62.9, 33.2, 18.7, 18.6. HRMS m/z: 362.14615, C16H20N5O5 362.14644.

PATENT
WO-2022047065-A2
PATENT
Example 1. (3aR, 4R, 6R, 6aR)-4-(4-aminopyrrole[2,1-f][1,2,4]triazin-7-yl)-6-(hydroxymethyl- Synthesis of 2,2-dimethyltetrahydrofuran[3,4-d][1,3]dioxolyl-4-carbonitrile (compound 5) |
Dissolve 5.62g of compound 69-0 in 30mL of acetone, then add 11.50mL of 2,2-dimethoxypropane and 1.34mL of 98% sulfuric acid, stir at 45°C for half an hour, cool to room temperature, and remove by rotary evaporation Organic solvents. Extract with 100 mL of ethyl acetate and 100 mL of saturated sodium bicarbonate solution. Repeat the extraction three times. Combine the ethyl acetate layers, add anhydrous sodium sulfate to dry, and filter to remove sodium sulfate. The organic solvent was removed by rotary evaporation, and 6.20 g of compound 5 (white solid, yield 97%) was obtained through column chromatography (eluent: petroleum ether/ethyl acetate (v/v) = 1/2). The hydrogen spectrum of the obtained compound 5 was detected, and the results are as follows: |
氢谱:1H NMR(400MHz,Chloroform-d)δ7.95(s,1H),7.11(d,J=4.7Hz,1H),6.69(dd,J=4.8,2.4Hz,1H),5.77(s,2H),5.42(d,J=6.6Hz,1H),5.24(dd,J=6.6,2.4Hz,1H),4.67(q,J=1.9Hz,1H),3.99(dd,J=12.5,1.9Hz,1H),3.84(dd,J=12.5,1.7Hz,1H),1.81(s,3H),1.40(s,3H)。 |
Example 2. ((3aR,4R,6R,6aR)-6-(4-aminopyrrole[2,1-f][1,2,4]triazin-7-yl)-6-cyano-2 ,Synthesis of 2-dimethyltetrahydrofuran[3,4-d][1,3]dioxol-4-yl)methylisobutyrate (compound INT-1) |
Dissolve 1.50g of compound 5 in 15ml of dichloromethane, then add 0.42mL of isobutyric acid and 55.40mg of 4-dimethylaminopyridine, stir for 10 minutes, add 1.02g of dicyclohexylcarbodiimide, and stir at room temperature. Stir for 24h. After column chromatography separation (eluent: petroleum ether/ethyl acetate (v/v) = 1/1), 1.71 g of compound INT-1 (white solid, yield 94%) was obtained. The hydrogen spectrum and carbon spectrum of the obtained compound INT-1 were detected, and the results are as follows: |
Hydrogen spectrum: 1 H NMR (400MHz, CDCl 3 ,ZQF-RD01-2)δ(ppm):7.99(s,1H),6.99(d,J=4.6Hz,1H),6.62(d,J=4.6Hz,1H),5.72(br,2H),5.49(d,J=6.8Hz,1H),4.93-4.90(dd,J=6.8Hz,4.3Hz,1H),4.61-4.58(q,J=4.4Hz,1H),4.44-4.26(m,2H),2.61-2.50(m,1H),1.77(s,3H),1.42(s,3H),1.17-1.14(q,J=3.8Hz,6H)。 |
Carbon spectrum: 13 C NMR (100MHz, CDCl 3 ,ZQF-RD01-2)δ(ppm):176.7,155.2,147.3,123.5,117.2,116.7,115.6,112.6,100.0,83.8,83.0,82.0,81.4,63.1,33.8,26.4,25.6,18.9。 |
PATENT
WO2023167938

To a solution of (3aR,4R,6R,6aR)-4-(4-aminopyrrolo[2,1-f][1,2,4]triazin-7-yl)-6-(hydroxymethyl)-2,2-dimethyltetrahydrofuro[3,4-d][1,3]dioxole-4-carbonitrile, Intermediate 1 (2000 mg, 6.0 mmol) (Siegel et. al. J. Med. Chem.2017, 60, 1648-1661) in tetrahydrofuran (THF) was added N,N-dimethylaminopyridine (DMAP) (0.03 eq). To the reaction mixture, isobutyric anhydride (1.1 eq) was added slowly. After the completion of the staring material, the reaction mixture was concentrated and purified by flash chromatography using 20% methanol in DCM as an eluant to give ((3aR,4R,6R,6aR)-6-(4- aminopyrrolo[2,1-f][1,2,4]triazin-7-yl)-6-cyano-2,2-dimethyltetrahydrofuro[3,4-d][1,3]dioxol-4- yl)methyl isobutyrate, Intermediate 1a. LCMS: MS m/z: 402.2 (M+1).
Concentrated HCl (5 eq, 1mL) was added to a solution of Intermediate 1a (1000 mg) in acetonitrile (10 mL), which was then stirred at room temperature for 2 hours. LCMS showed the product formation. The reaction was stopped after 4 hours. The reaction mixture was diluted with ethyl acetate and quenched with saturated bicarbonate. The organic layer was separated, washed with brine, dried over sodium sulphate, and concentrated. The residue was purified by flash chromatography using 30% methanol DCM as an eluant. The collected fractions were concentrated to give Intermediate 2.1H NMR (400 MHz, Methanol-d4) δ 7.88 (s, 1H), 6.96 – 6.85 (m, 2H), 4.50 – 4.27 (m, 4H), 4.16 (dd, J = 6.2, 5.3 Hz, 1H), 2.56 (p, J = 7.0 Hz, 1H), 1.14 (dd, J = 7.0, 3.8 Hz, 6H); LCMS: MS m/z: 362.1 (M+1).


AS ON DEC2021 3,491,869 VIEWS ON BLOG WORLDREACH AVAILABLEFOR YOUR ADVERTISEMENT

join me on Linkedin
Anthony Melvin Crasto Ph.D – India | LinkedIn
join me on Researchgate
RESEARCHGATE
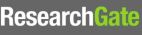
join me on Facebook
Anthony Melvin Crasto Dr. | Facebook
join me on twitter
Anthony Melvin Crasto Dr. | twitter
+919321316780 call whatsaapp
EMAIL. amcrasto@gmail.com
//////////
Names | |
---|---|
IUPAC name[(2R,3S,4R,5R)-5-(4-Aminopyrrolo[2,1-f] [1,2,4]triazin-7-yl)-5-cyano-3,4-dihydroxyoxolan-2-yl]methyl 2-methylpropanoate | |
Identifiers | |
CAS Number | 2647441-36-7 |
3D model (JSmol) | Interactive image |
ChEMBL | ChEMBL4863829 |
ChemSpider | 115037299 |
PubChemCID | 162513664 |
UNII | Q55KCM7PXB |
showInChI | |
showSMILES | |
Properties | |
Chemical formula | C16H19N5O5 |
Molar mass | 361.358 g·mol−1 |
Except where otherwise noted, data are given for materials in their standard state (at 25 °C [77 °F], 100 kPa). |
References
- ^ “Clinical Trials Register”.
- ^ “Gilead touts Veklury resilience against mutated coronavirus, plots phase 3 for new COVID oral antiviral”. 19 October 2022.
- ^ Cho, A.; Saunders, O. L.; Butler, T.; Zhang, L.; Xu, J.; Vela, J. E.; Feng, J. Y.; Ray, A. S.; Kim, C. U. (2012). “Synthesis and antiviral activity of a series of 1′-substituted 4-aza-7,9-dideazaadenosine C-nucleosides”. Bioorganic & Medicinal Chemistry Letters. 22 (8): 2705–2707. doi:10.1016/j.bmcl.2012.02.105. PMC 7126871. PMID 22446091.
- ^ Yan, Victoria C.; Muller, Florian L. (2020). “Advantages of the Parent Nucleoside GS-441524 over Remdesivir for Covid-19 Treatment”. ACS Medicinal Chemistry Letters. 11 (7): 1361–1366. doi:10.1021/acsmedchemlett.0c00316. PMC 7315846. PMID 32665809.
- ^ Gordon, C. J.; Tchesnokov, E. P.; Woolner, E.; Perry, J. K.; Feng, J. Y.; Porter, D. P.; Götte, M. (2020). “Remdesivir is a direct-acting antiviral that inhibits RNA-dependent RNA polymerase from severe acute respiratory syndrome coronavirus 2 with high potency”. The Journal of Biological Chemistry. 295 (20): 6785–6797. doi:10.1074/jbc.RA120.013679. PMC 7242698. PMID 32284326.
- ^ Cao, Liu; et al. (2022). “The adenosine analog prodrug ATV006 is orally bioavailable and has preclinical efficacy against parental SARS-CoV-2 and variants”. Science Translational Medicine. 14 (661): eabm7621. doi:10.1126/scitranslmed.abm7621. PMC 9161374. PMID 35579533.
- ^ “Espacenet – search results”.
//////////Obeldesivir, GS-5245, ATV006
CC(C)C(OC[C@H]1O[C@@](C#N)(C2=CC=C3C(N)=NC=NN32)[C@H](O)[C@@H]1O)=O
Oditrasertib
Oditrasertib
Molecular Formula | C14H15F2N3O2 |
Molecular Weight | 295.2846 |
- UNII-I1YQT8HC89
- I1YQT8HC89
- cas 2252271-93-3
- 4-(3,3-Difluoro-2,2-dimethylpropanoyl)-3,5-dihydro-2H-pyrido(3,4-F)(1,4)oxazepine-9-carbonitrile
PYRIDO(3,4-F)-1,4-OXAZEPINE-9-CARBONITRILE, 4-(3,3-DIFLUORO-2,2-DIMETHYL-1-OXOPROPYL)-2,3,4,5-TETRAHYDRO-
Oditrasertib is a serine/threonine kinase (STK) inhibitor.
PATENT
[WO2018213632A1]
https://patentscope.wipo.int/search/en/detail.jsf?docId=WO2018213632



AS ON DEC2021 3,491,869 VIEWS ON BLOG WORLDREACH AVAILABLEFOR YOUR ADVERTISEMENT

join me on Linkedin
Anthony Melvin Crasto Ph.D – India | LinkedIn
join me on Researchgate
RESEARCHGATE
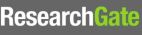
join me on Facebook
Anthony Melvin Crasto Dr. | Facebook
join me on twitter
Anthony Melvin Crasto Dr. | twitter
+919321316780 call whatsaapp
EMAIL. amcrasto@gmail.com
//////////
///////////Oditrasertib,
CC(C)(C(F)F)C(=O)N1CCOC2=C(C=NC=C2C1)C#N
ORZILOBEN
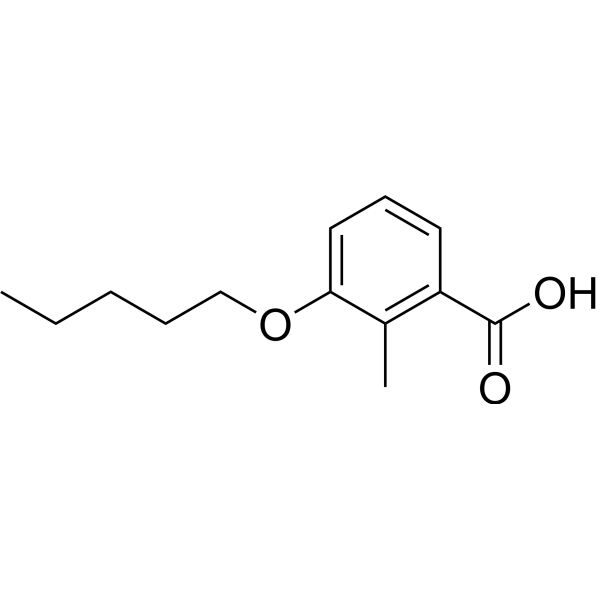
ORZILOBEN,
CAS 1555822-28-0
2-methyl-3-(pentyloxy)benzoic acid
Molecular Weight | 222.28 |
---|---|
Formula | C13H18O3 |
Orziloben is a medium chain fatty acid (MCFA) analogue[1].
Patent
WO2020074964
The rising global epidemic of obesity and its comorbidities, e.g., type 2 diabetes mellitus and hyperlipidemia, is placing an enormous burden both on public health (mortality and morbidity) and on the available public health resources required to treat these conditions.
Current drugs that treat hyperlipidemia (e.g., statins, omega-3 fatty acids, fibrates) have mostly neutral effects on glycemic control, whilst drugs targeting glycemic control e.g., insulin, thiazolidinediones (TZDs), have adverse effects upon bodyweight and (for TZDs) other unwanted side-effects restricting their use.
In addition to hyperlipidemia and type 2 diabetes, a marked increase in the prevalence of non-alcoholic fatty liver disease (NAFLD) has occurred. NAFLD has become the most common chronic liver condition in Western populations in relation to the obesity and type 2 diabetes epidemics. The prevalence of non-alcoholic steatohepatitis (NASH), a form of NAFLD that is associated with hepatic inflammation and ballooning of hepatocytes, is expected to increase by 63% between 2015 and 2030 in the United States (Estes, Hepatology, 2018; 67(1): 123-133), where NASH is expected to become the leading cause of liver transplantation by 2020. As liver fibrosis, but not inflammation, is associated with mortality and morbidity in NASH patients, drugs which prevent progression/induce regression of fibrosis are also a focus of biomedical research.
The development of novel compounds that simultaneously target both hyperlipidemia and glycemic control, without the adverse side-effects (e.g., weight gain) typically associated with insulin sensitising drugs is thus a desirable goal. Such compounds would be even more attractive if they could additionally prevent the progression/reverse hepatic fibrosis and reduce hepatic steatosis. The present invention addresses these needs for new treatment methods, compounds, and pharmaceutical compositions.



AS ON DEC2021 3,491,869 VIEWS ON BLOG WORLDREACH AVAILABLEFOR YOUR ADVERTISEMENT

join me on Linkedin
Anthony Melvin Crasto Ph.D – India | LinkedIn
join me on Researchgate
RESEARCHGATE
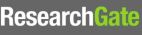
join me on Facebook
Anthony Melvin Crasto Dr. | Facebook
join me on twitter
Anthony Melvin Crasto Dr. | twitter
+919321316780 call whatsaapp
EMAIL. amcrasto@gmail.com
//////////
/////////ORZILOBEN, 1555822-28-0, OBESITY
O=C(C1=C(C)C(OCCCCC)=CC=C1)O
Opevesostat
Opevesostat
- ODM208
2231294-96-3
Chemical Formula: C21H26N2O5S
Molecular Weight: 418.508
2-[(1,3-dihydro-2H-isoindol-2-yl)methyl]-5-{[1-(methanesulfonyl)piperidin-4-yl]methoxy}-4H-pyran-4-one
Opevesostat tosylate |
4H-Pyran-4-one, 2-[(1,3-dihydro-2H-isoindol-2-yl)methyl]-5-[[1-(methylsulfonyl)-4-piperidinyl]methoxy]-, 4-methylbenzenesulfonate (1:1)
2-((1,3-DIHYDRO-2H-ISOINDOL-2-YL)METHYL)-5-((1-(METHYLSULFONYL)-4-PIPERIDINYL)METHOXY)-4H-PYRAN-4-ONE, TOSYLATE
useful in the treatment of a steroid receptor, in particular androgen receptor (AR), dependent conditions and diseases, and to pharmaceutical compositions containing such compounds.
Prostate cancer is worldwide the most common cancer in men. Even though the 5-year survival rate of patients with localized prostate cancer is high, the prognosis for those patients, who develop castration-resistant prostate cancer (CRPC) within that 5-year follow-up period, is poor.
The androgen receptor (AR) signalling axis is critical in all stages of prostate cancer. In the CPRC stage, disease is characterized by high AR expression, AR amplification and persistent activation of the AR signalling axis by residual tissue/tumor androgens and by other steroid hormones and intermediates of steroid biosynthesis. Thus, treatment of advanced prostate cancer involves androgen deprivation therapy (ADT) such as hormonal manipulation using gonadotropin-releasing hormone (GnRH) agonists/antagonists or surgical castration, AR antagonists or CYP17A1 inhibitors (such as abiraterone acetate in combination with prednisone).
Although therapies can initially lead to disease regression, eventually majority of the patients develop a disease that is refractory to currently available therapies. Increased progesterone levels in patients treated with abiraterone acetate has been hypothesized to be one of the resistance mechanisms. Several nonclinical and clinical studies have indicated upregulation of enzymes that catalyse steroid biosynthesis at the late stage of CRPC. Very recently it has been published that 11β-OH androstenedione can be
metabolized into 11-ketotestosterone (11-K-T) and 11-ketodehydrotestosterone (11-K-DHT) which can bind and activate AR as efficiently as testosterone and dihydrotestosterone. It has been shown that these steroids are found in high levels in plasma and tissue in prostate cancer patients, suggesting their role as AR agonists in CRPC. Furthermore, it has been addressed that prostate cancer resistance to CYP17A1 inhibition may still remain steroid dependent and responsive to therapies that can further suppress de novo intratumoral steroid synthesis upstream of CYP17A1, such as by CYP11A1 inhibition therapy (Cai, C. et al, Cancer Res., 71(20), 6503-6513, 2011).
Cytochrome P450 monooxygenase 11A1 (CYP11A1), also called cholesterol side chain cleavage enzyme, is a mitochondrial monooxygenase which catalyses the conversion of cholesterol to pregnenolone, the precursor of all steroid hormones. By inhibiting CYP11A1, the key enzyme of steroid biosynthesis upstream of CYP17A1, the total block of the whole steroid biosynthesis can be achieved. CYP11A1 inhibitors may therefore have a great potential for treating steroid hormone dependent cancers, such as prostate cancer, even in advanced stages of the disease, and especially in those patients who appear to be hormone refractory. It has been recently shown that a compound having CYP11A1 inhibitory effect significantly inhibited tumor growth in vivo in a murine CRPC xenograft model (Oksala, R. et al, Annals of Oncology, (2017) 28 (suppl.
PATENT
WO2018115591
https://patentscope.wipo.int/search/en/detail.jsf?docId=WO2018115591&_cid=P20-LQXJT7-60871-1
Example 4. SIMILAR
N-((4-(((6-(Isoindolin-2-ylmethyl)-4-oxo-4H-pyran-3-yl)oxy)methyl)cyclohexyl)- methyl)methanesulfonamide (Compound 173)
To a solution of 5-hydroxy-2-(isoindolin-2-ylmethyl)-4H-pyran-4-one (0.10 g, 0.41 mmol) in DMF (2 ml) were added (4-(methylsulfonamidomethyl)cyclohexyl)methyl methanesulfonate (0.14 g, 0.45 mmol) and K2CO3 (0.12 g, 0.8 mmol). The reaction mixture was heated at 80 °C for 2 h. The mixture was cooled to RT, water (10 ml) was added and the product was extracted with EtOAc. The combined extracts were washed with water, dried with Na2SO4, filtered and evaporated. The crude product was purified by column chromatography to afford the title compound (0.06 g). 1H NMR (400 MHz, Chloroform-d) δ ppm 0.92 – 1.11 (m, 4 H) 1.40 – 1.63 (m, 2 H) 1.78 – 2.00 (m, 4 H) 2.91 – 2.99 (m, 5 H) 3.65 (d, J=6.46 Hz, 2 H) 3.77 (s, 2 H) 4.03 (s, 4 H) 5.04 (br t, J=6.31 Hz, 1 H) 6.49 (s, 1 H) 7.20 (s, 4 H) 7.59 (s, 1 H).
ntermediate 58: 5-Hydroxy-2-(isoindolin-2-ylmethyl)-4H-pyran-4-one
To a stirred solution of 2-(chloromethyl)-5-hydroxy-4H-pyran-4-one (2.0 g, 12.5 mmol) in acetonitrile (50 mL) were added DIPEA (3.22 mL, 25.0 mmol) and isoindoline (1.78 g, 25.0 mmol) at RT. When the reaction was complete, the precipitated solid was filtered and washed with EtOAc. The title compound was collected as pale brown solid (1.1 g). LC-MS: m/z 244.1 (M+H)+.
///////////////////


AS ON DEC2021 3,491,869 VIEWS ON BLOG WORLDREACH AVAILABLEFOR YOUR ADVERTISEMENT

join me on Linkedin
Anthony Melvin Crasto Ph.D – India | LinkedIn
join me on Researchgate
RESEARCHGATE
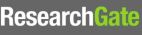
join me on Facebook
Anthony Melvin Crasto Dr. | Facebook
join me on twitter
Anthony Melvin Crasto Dr. | twitter
+919321316780 call whatsaapp
EMAIL. amcrasto@amcrasto
//////////
/////////Opevesostat, ODM 208
O=C1C=C(CN2CC3=C(C=CC=C3)C2)OC=C1OCC4CCN(S(=O)(C)=O)CC4
Sotuletinib HCl

Sotuletinib HCl
CAS: 2222138-31-8 (HCl)
Chemical Formula: C20H23ClN4O3S
Molecular Weight: 434.939
7W3V82OQ0P
Synonym: Sotuletinib HCl; Sotuletinib hydrochloride, Sotuletinib monohydrochloride, BLZ945; BLZ 945; BLZ-945;
IUPAC/Chemical Name: 4-((2-(((1R,2R)-2-hydroxycyclohexyl)amino)benzo[d]thiazol-6-yl)oxy)-N-methylpicolinamide hydrochloride
2-PYRIDINECARBOXAMIDE, 4-((2-(((1R,2R)-2-HYDROXYCYCLOHEXYL)AMINO)-6-BENZOTHIAZOLYL)OXY)-N-METHYL- |
Sotuletinib, also known as BLZ945, is a potent and selective CSF-1R kinase inhibitor. BLZ945 showed effects of CSF1R inhibition on other tumor-infiltrating immune cells. BLZ945 attenuates the turnover rate of TAMs while increasing the number of CD8+ T cells that infiltrate cervical and breast carcinomas. BLZ945 decreases the growth of malignant cells in the mouse mammary tumor virus-driven polyomavirus middle T antigen (MMTV-PyMT) model of mammary carcinogenesis. BLZ945 prevents tumor progression in the keratin 14-expressing human papillomavirus type 16 (K14-HPV-16) transgenic model of cervical carcinogenesis.
Sotuletinib (BLZ945) is an experimental drug in development for the treatment of amyotrophic lateral sclerosis (ALS). It works as a colony-stimulating factor 1 (CSF1) receptor inhibitor.[1][2][3]
- OriginatorCelgene Corporation; Novartis
- ClassAmides; Amines; Antineoplastics; Benzothiazoles; Cyclohexanols; Ethers; Pyridines; Small molecules
- Mechanism of ActionMacrophage colony stimulating factor receptor antagonists
- Phase IIAmyotrophic lateral sclerosis
- Phase I/IISolid tumours
- 05 Dec 2022Novartis Pharmaceuticals terminates a phase I/II trials in Solid tumours (Combination therapy, Late-stage disease, Metastatic disease) in Taiwan, Japan, Israel (PO) in US, Israel, Italy, Japan, Singapore, Spain, Taiwan and Switzerland (EudraCT2015-005806-12) (NCT02829723)
- 14 Feb 2022Adverse events and pharmacodynamics data from preclinical macaque model study in brain disorders presented at the 29th Conference on Retroviruses and Opportunistic Infections
- 03 Dec 2020Chemical structure information added
An orally bioavailable inhibitor of colony stimulating factor 1 receptor (CSF-1R; CSF1R), with potential antineoplastic activity. CSF1R inhibitor BLZ945 selectively binds to CSF1R expressed on tumor-associated macrophages (TAMs), blocks the activity of CSF1R, and inhibits CSF1R-mediated signal transduction pathways. This inhibits the activity and proliferation of TAMs, and reprograms the immunosuppressive nature of existing TAMs. Altogether, this reduces TAM-mediated immune suppression in the tumor microenvironment, re-activates the immune system, and improves anti-tumor cell responses mediated by T-cells. CSF1R, also known as macrophage colony-stimulating factor receptor (M-CSFR) and CD115 (cluster of differentiation 115), is a cell-surface receptor for its ligand, colony stimulating factor 1 (CSF1); this receptor is overexpressed by TAMs in the tumor microenvironment, and plays a major role in both immune suppression and the induction of tumor cell proliferation.
PATENT
The free base and salts of the compound of formula (I) may be prepared for example, according to the procedures given in International Patent Application No. PCT/US2007/066898 filed on Apr. 18, 2007 and published as WO2007/121484 on Oct. 25, 2007. The compound of formula (I) has the chemical name: 4-(2-((1R,2R)-2-hydroxycyclohexylamino)benzothiazol-6-yloxy)-N-methylpicolinamide and is also known as BLZ945.








Step 1. Preparation of 4-(2-((lR,2R)-2-aminocyclohexylamino)benzo[d]thiazol-6-yloxy)-N-methylpicolinamide
To the solution of N-methyl-4-(2-(methylsulfinyl)benzo[d]thiazol-6-yloxy)picolinamide (15 mg, 43 μmole) in 400 μL of NMP was added (lR,2R)-cyclohexane-1,2-diamine (17 mg, 150 μmole). The reaction solution was stirred at 105°c for 24 hours. The crude reaction solution was purified on prep HPLC and evaporated in vaccuo to give 4-(2-((lR,2R)-2-aminocyclohexylamino)benzo[d]thiazol-6-yloxy)-N-methylpicolinamide (12 mg, 30 μmole) as white powder. ES/MS m/z 398.1(MH+).
PATENT
https://patents.google.com/patent/US20200093801A1
PATENT
CN116139135
PATENT
US20200190057
PATENT
CN110475555


AS ON DEC2021 3,491,869 VIEWS ON BLOG WORLDREACH AVAILABLEFOR YOUR ADVERTISEMENT

join me on Linkedin
Anthony Melvin Crasto Ph.D – India | LinkedIn
join me on Researchgate
RESEARCHGATE
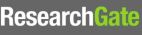
join me on Facebook
Anthony Melvin Crasto Dr. | Facebook
join me on twitter
Anthony Melvin Crasto Dr. | twitter
+919321316780 call whatsaapp
EMAIL. amcrasto@amcrasto
//////////
///////////
References
- ^ Pognan, François; Buono, Chiara; Couttet, Philippe; Galarneau, Jean-René; Timsit, Yoav; Wolf, Armin (29 October 2022). “Liver enzyme delayed clearance in rat treated by CSF1 receptor specific antagonist Sotuletinib”. Current Research in Toxicology. 3: 100091. doi:10.1016/j.crtox.2022.100091. ISSN 2666-027X.
- ^ Thongchot, Suyanee; Duangkaew, Supani; Yotchai, Wasan; Maungsomboon, Sorranart; Phimolsarnti, Rapin; Asavamongkolkul, Apichat; Thuwajit, Peti; Thuwajit, Chanitra; Chandhanayingyong, Chandhanarat (2 December 2022). “Novel CSF1R-positive tenosynovial giant cell tumor cell lines and their pexidartinib (PLX3397) and sotuletinib (BLZ945)-induced apoptosis”. Human Cell. 36 (1): 456–467. doi:10.1007/s13577-022-00823-0.
- ^ Martinez-Gonzalez, Loreto; Martinez, Ana (1 February 2023). “Emerging clinical investigational drugs for the treatment of amyotrophic lateral sclerosis”. Expert Opinion on Investigational Drugs. 32 (2): 141–160. doi:10.1080/13543784.2023.2178416.
////////Sotuletinib HCl, BLZ945, BLZ 945, BLZ-945,
O=C(NC)C1=NC=CC(OC2=CC=C3N=C(N[C@H]4[C@H](O)CCCC4)SC3=C2)=C1.[H]Cl
TACACICLIB, AUR-102, AURIGENE
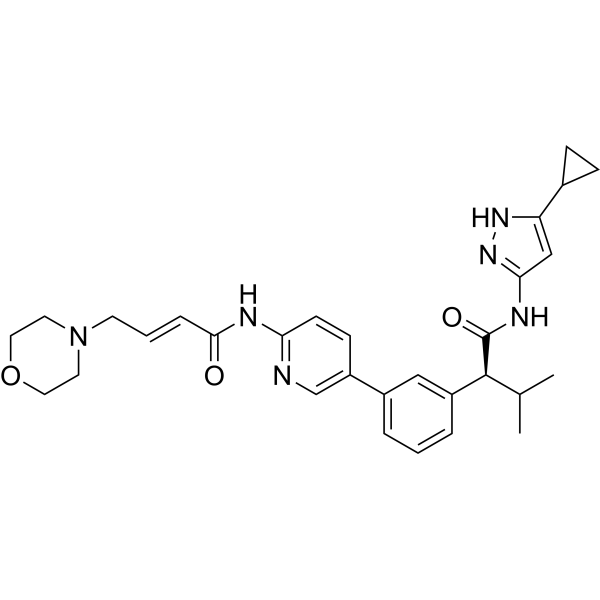
Tacaciclib
2768774-66-7
AUR-102
- Tacaciclib
- SCHEMBL24548621
- GTPL12880
- 528.6 g/mol
- C30H36N6O3
INN 12755
UNI D3G4JKK1MA
(2S)-N-(5-cyclopropyl-1H-pyrazol-3-yl)-3-methyl-2-[3-[6-[[(E)-4-morpholin-4-ylbut-2-enoyl]amino]pyridin-3-yl]phenyl]butanamide
(αS)-N-(5-Cyclopropyl-1H-pyrazol-3-yl)-α-(1-methylethyl)-3-[6-[[(2E)-4-(4-morpholinyl)-1-oxo-2-buten-1-yl]amino]-3-pyridinyl]benzeneacetamide
Benzeneacetamide, N-(5-cyclopropyl-1H-pyrazol-3-yl)-α-(1-methylethyl)-3-[6-[[(2E)-4-(4-morpholinyl)-1-oxo-2-buten-1-yl]amino]-3-pyridinyl]-, (αS)-
Tacaciclib is a CDK inhibitor, antineoplastic effect.
The present invention is directed to methods of preparation of compound of formula (I) that is useful for inhibiting Cyclin-dependent kinase 7 (CDK7) and for treating diseases or disorders mediated thereby.
CDK7, which complexes with cyclin H and RING-finger protein MAT1, phosphorylates the cell cycle CDKs in the activation of T-loop, to promote their activities (Fisher et al., Cell., Aug 26;78(4):713- 24, 1994). As such, it has been proposed that inhibiting CDK7 would provide a potent means of inhibiting cell cycle progression, which may be especially relevant given that there is compelling evidence from gene knockout studies in mice for lack of an absolute requirement for CDK2, CDK4 and CDK6 for the cell cycle at least in most cell types (M alumbres et al., Nature Cell Biology, 11, 1275 – 1276, 2009), whilst different tumors appear to require some, but they are independent of other interphase CDKs (CDK2, CDK4 , CDK6). Recent genetic and biochemical studies have confirmed the importance of CDK7 for cell cycle progression (Larochelle. et al., Mol Cell., Mar 23;25(6):839-50. 2007; Ganuza et al., EM BO J., May 30; 31(11): 2498-510, 2012).
Cyclin-dependent kinase 7 (CDK7) activates cell cycle CDKs and is a member of the general Transcription factor II Human (TFIIH). CDK7 also plays a role in transcription and possibly in DNA repair. The trimeric Cak complex CDK7/CyclinH/MATl is also a component of TFIIH, the general transcription/DNA repair factor IIH (Morgan, DO., Annu.Rev. Cell Dev. Biol. 13, 261-91, 1997). As a TFIIH subunit, CDK7 phosphorylates the CTD (Carboxy-Terminal-Domain) of the largest subunit of RNA polymerase II (pol II). The CTD of mammalian pol (II) consists of 52 heptad repeats with the consensus sequence 1 YSPTSPS 7 and the phosphorylation status of the Ser residues at positions 2 and 5 has been shown to be
important in the activation of RNAP-II indicating that it is likely to have a crucial role in the function of the CTD. CDK7, which primarily phosphorylates Ser-5 (PSS) of RNAP-II at the promoter as part of transcriptional initiation (Gomes et ah, Genes Dev. 2006 Mar 1; 20(5):601-12, 2006), in contrast with CDK9, which phosphorylates both Ser-2 and Ser-5 of the CTD heptad (Pinhero et al., Eur. J. Biochem., 271, pp. 1004-1014, 2004).
In addition to CDK7, other CDKs have been reported to phosphorylate and regulate RNA pol (II) CTD. The other CDKs include, Cdk9/ Cyclin T1 or T2 that constitute the active form of the positive transcription elongation factor (P-TEFb) (Peterlin and Price, Mol Cell., Aug 4; 23(3): 297-305,2006) and Cdkl2/Cyclin K and Cdkl3/Cyclin K as the latest members of RNAPII CTD kinases (Bartkowiak et al., Genes Dev., Oct 1 5;24(20):2303-16, 2010; Blazek et al., Genes Dev .Oct 15;25(20):2158-72, 2011).
Disruption of RNAP II CTD phosphorylation has been shown to preferentially effect proteins with short half-lives, including those of the anti-apoptotic BCL-2 family. (Konig et al., Blood, 1, 4307-4312, 1997; The transcriptional non-selective cyclin-dependent kinase inhibitor flavopiridol induces apoptosis in multiple myeloma cells through transcriptional repression and down-regulation of Mcl-1; (Gojoet al., Clin. Cancer Res. 8, 3527-3538, 2002).
This suggests that the CDK7 enzyme complexes are involved in multiple functions in the cell: cell cycle control, transcription regulation and DNA repair. It is surprising to find one kinase involved in such diverse cellular processes, some of which are even mutually exclusive. It also is puzzling that multiple attempts to find cell cycle dependent changes in CDK7 kinase activity remained unsuccessful. This is unexpected since activity and phosphorylation state of its substrate, CDC2, fluctuate during the cell cycle. In fact, it is shown that cdk7 activity is required for the activation of both Cdc2/Cyclin A and Cdc2/Cyclin B complexes, and for cell division. (Larochelle, S. et al. Genes Dev 12,370-81, 1998). Indeed, flavopiridol, a non-selective pan-CDK inhibitor that targets CTD kinases, has demonstrated efficacy for the treatment of chronic lymphocytic leukemia (CLL), but suffers from a poor toxicity profile (Lin et al.,). 27, 6012-6018, 2009; Christian et al., Clin. Lymphoma Myeloma, 9, Suppl.
3, S179-S185, 2009).
International publication WO2016193939, which is incorporated herein by reference for all purposes describes CDK7 inhibitors and processes for the preparation thereof. Inhibitors of CDK7 are currently being developed for the treatment of cancer. For drug development, it is typically advantageous to employ individual stereoisomers as they exhibit marked differences in pharmacodynamic, pharmacokinetic, and toxicological properties.
PATENT
WO 2016/193939 COMPD 44
https://patents.google.com/patent/WO2016193939A1/en
InventorSusanta SamajdarRamulu PoddutooriChetan PanditSubhendu MUKHERJEERajeev Goswami
AURIGENE DISCOVERY TECHNOLOGIES LIMITED [IN]/[IN]
Inventors
- SAMAJDAR, Susanta
- PODDUTOORI, Ramulu
- PANDIT, Chetan
- MUKHERJEE, Subhendu
- GOSWAMI, Rajeev
PATENT
Applicants
- AURIGENE ONCOLOGY LIMITED [IN]/[IN]
Inventors
- PODDUTOORI, Ramulu
- VIJAYKUMAR BHAT, Uday
- THIMMASANDRA SEETHAPPA, Devaraja
WO2022229835
Example- 1: Preparation of compound of formula (I)
Scheme-1: Preparation of KRM-A


Step-4
KRM-A 4
Step-1: Preparation of 2-(3-bromophenyl)-3-methylbutanoic acid (1)
2M LDA (698 mL, 1.38mol) was added to a solution of 2-(3-bromophenyl) acetic acid (XA, 150 g, 0.69 mol) in THF (700mL) at -78 °C over a period of 30 min. The reaction mixture was stirred for 2h at -78 °C followed by a drop wise addition of isopropyl bromide (X B , 255 g, 2.07 mol) over a period of 30 min. The reaction mixture was stirred at room temperature overnight. Then, the reaction mixture was quenched with IN HC1 (pH 2) and the obtained product was extracted to ethyl acetate (500 mL x 3). The combined organic layer was washed with water followed by brine solution. The organic layer was dried over anhydrous sodium sulfate and concentrated under reduced pressure to afford the crude compound which was purified by silica column by eluting with 0-10% ethyl acetate-hexane system to afford the title compound (150 g, 83% yield) , HPLC purity-96%. The compound of formula (1) can also be prepared by the procedure described in CN 110590747.
Step-2: Preparation of Compound 3
2-(3-bromophenyl)-3-methylbutanoic acid (1, 510 g, 1.98 mol) was dissolved in 30% of IP A in water (10.2 L; 3.06 L of IPA-7.14 L of water) and ( 1L\ 2i ?)-cyclohexane-1,2-diamine (2, 113 g, 0.9 mol) was added. The reaction mixture was stirred at room temperature for 10 min until the precipitation was observed, then was heated to 100 °C until the solution became clear and stirred at the same temperature for another 30 min. The reaction mixture was allowed to slowly reach room temperature for 8-12h. The obtained solid was filtered and washed with 500 mL of 30% IPA-water mixture and dried under vacuum to afford the compound 3 (620 g, wet).
Work up (for Chiral purity): Small portion (100 mg) of compound 3 was taken in DCM (2-3 mL) and was added IN HC1 (pH 2) at 0 °C until the clear solution was observed. The compound was extracted into DCM, dried over NaiSCL and the solvent was evaporated to afford the title compound as white solid (20 mg). Chiral HPLC was recorded for this sample and 20.6% of undesired isomer was observed in chiral HPLC.
In order to improve the chiral purity of the title compound, the recrystallization method was performed as described below.
Step-3: Recrystallization
The compound 3 (619.90 g) was taken in 30% of IP A in water (12.4 L), then the mixture was heated to 100 °C until the solution became clear and was stirred at the same temperature for another 30min. The reaction mixture was allowed to reach room temperature slowly for 8-12h.
The obtained solid was filtered and washed with 500mL 30% IPA-water and dried under vacuum to afford a desired compound (360g, wet).
Work up for analysis (for Chiral purity): Small portion (100 mg) from above compound was taken in DCM (2-3mL), was added IN HC1 (pH 2) at 0 °C until the clear solution was observed and the compound was extracted to DCM, dried over NaiSCL and the solvent was evaporated to afford title compound as white solid (35 mg). Chiral HPLC was recorded for this sample and 10.3% of undesired isomer was observed in chiral HPLC.
The recrystallization method was repeated for three more times by using 30% of IPA in water as per the aforesaid procedure to obtain the purity of greater than 98.50% ee along with 0.27% other isomer to afford 286 g of compound 4.
Step-4: Preparation of (S)-2-(3-bromophenyl)-3-methylbutanoic acid (KRM-A)
The compound 4 (286 g) was taken in DCM (1.3 L), then was added IN HC1 at 0 °C until the clear solution was observed, and the compound was extracted to DCM (500 mL x 2). The organic layer was separated, washed with brine solution (500 mL) and dried over NaiSCL. The solvent was evaporated from the reaction mixture to afford title compound as white solid (148 g, 60% yield). Chiral HPLC: 98.50%
*H NMR (400MHz, DMSO-de): d 12.5 (s, 1H), 7.50-7.44 (m, 2H), 7.34-7.26 (m, 2H), 3.16 (d, 1H), 2.23-2.11 (m, 1H), 0.98 (d, 3H), 0.63 (d, 3H); Chiral HPLC: 98.50% retention time: 4,588 min.
Scheme-2: Preparation of compound of formula (I)


Step-1: Synthesis of (S)-2-(3-bromophenyl)-N-(5-cyclopropyl-1H-pyrazol-3-yl)-3-methylbutanamide
Step-la: Preparation ofKRM-D
To a stirred solution of KRM-A (lOOg, O.388mol) in dry DCM (600 mL, 6 vol), a catalytic amount of DMF (10 mL) was added followed by oxalyl chloride (45 mL, 0.525 mol) dropwise at 0°C over a period of 30 min. After completion of addition, the reaction mixture was stirred for 15 min at the same temperature. The reaction mixture was allowed to reach room temperature and stirred for 2 to 4h. After completion of the reaction (reaction was monitored by TLC, acid chloride formation was checked by quenching an aliquot of reaction mixture with MeOH), the reaction mixture was concentrated under vacuum at 40°C-45°C to afford crude (S)- 2-(3-bromophenyl)-3-methylbutanoyl chloride (KRM-D). The crude KRM-D was dissolved in toluene (500mL) and used for next step.
Step-lb: Preparation of compound of formula (II)
(5)-2-(3-bromophcnyl)-3-mcthylbutanoyl chloride in toluene was added slowly to a pre-cooled solution (0 to 5 °C) of ieri-butyl 3-amino-5-cyclopropyl-1H-pyrazole- l-carboxylate (KRM-B, 95.5g, 0.427 mol) and N, N-diisopropylethyl amine (100 mL, 0.583 mol) in toluene (1.2 L) at 0 °C for the period of l-2h. The reaction mixture was allowed to reach RT and stirred overnight. The reaction mixture was then cooled to 0-5°C and washed with ice-cold 1.5N HC1 (3 x 500 mL). The organic layer was washed with sodium bicarbonate solution (500 mL), brine solution (500 mL), dried over anhydrous NaiSCL , filtered and concentrated under vacuum at 45-50°C to afford crude tert-butyl (S)-5-( 2-(3-bromophenyl)-3-methylbutanamido)-3-
cyclopropyl- lH-pyrazole- 1-carboxylate (compound of formula (IG)) as light brown oil (~180g, LCMS: m/z= 461.9 (M+H) + , HPLC: 80.80%, retention time:15.89 min) . The crude product was taken as such for next step without further purification.
Step-1 c: Preparation of compound of formula (I)
To a suspension of tert-butyl (S)-5-(2-(3-bromophenyl)-3-methylbutanamido)-3-cyclopropyl-1H-pyrazole-1-carboxylate (180 g, 1,731 mol) in dioxane (360 mL ) was added 2N aqueous HC1 (360 mL) at 0 °C. The reaction mixture was stirred overnight at room temperature. After completion of the reaction, dioxane was concentrated, and the reaction mixture was diluted with water (500 mL) and basified with solid sodium bicarbonate (until pH-8). The obtained compound was extracted with DCM (700 mL x 3). The combined organic layers were washed with water (300 mL), brine solution (300 mL), and dried over anhydrous NaiSCL . The organic layer was concentrated to obtain a crude (S)-2-(3-bromophenyl)-N-(5-cyclopropyl-lH-pyrazol-3-yl)-3-methylbutanamide (Compound of formula (G)) as a semi-solid. The crude was dissolved in toluene (500 mL) and the solution was stirred for 18 h. The obtained solid was filtered and washed with toluene (100 mL) and n-heptane (200 mL). The solid was further dried under vacuum at 45-50°C for 6 h to afford a title compound (1 lOg, Yield: 78% over two steps). LCMS: m/z= 362 (M+H) + , HPLC: 97.66%, retention time: 24.10 min
Step-2: Preparation of (S, E)-N-(5-(3-(l-((5-cyclopropyl-lH-pyrazol-3-yl) amino)-3-methyl-l-oxobutan-2- yl) phenyl) pyridin-2-yl)-4-morpholinobut-2-enamide (Compound of formula (I))
To a degassed solution of (5)-2-(3-bromophcnyl)-N-(5-cyclopropyl-1 H-pyrazol-3-yl)-3-methylbutanamide (50 g, 0.138 mol) and (E)-4 -morpholino-N-(5-(4,4,5,5-tetramethyl-l,3,2-dioxaborolan-2-yl)pyridin-2-yl)but-2-enamide (KRM-C, 56.6 g, 0.151 mol, 1.1 eq) (prepared according to the procedure described in W02020202001) in 1,4-dioxane (500 mL, 10 vol) and water (100 mL, 2 vol) was added K3PO4 tribasic (73.2 g, 0.345 mol, 2.5 eq) at room temperature The reaction mass was stirred for 20 min with argon purging (degassing). Pd(dppf)Ch.DCM (3.38 g, 0.0042 mol, and 0.03eq) was added to the reaction mixture and the reaction mixture was heated to 90°C for 1-2 h (The reaction was monitored by TLC using 10% methanol in DCM as solvent system).
After completion of the reaction, the reaction mass was cooled to room temperature and filtered through Celite ® bed. The bed was washed with 1, 4-dioxane (200 mL) and the filtrate was concentrated to get crude compound. The crude compound was dissolved in 5% methanol in DCM (400 mL) and washed with water (200 mL x 2). The aqueous layer was separated and
extracted with DCM (100 mL x 2). The combined organic layer was washed with brine solution, filtered and dried over sodium sulfate. The organic layer was concentrated under vacuum at 35-40°C to obtain crude title compound (~80g).
The crude compound of formula (I), (80 g) was dissolved in 700 mL of ethyl acetate. The reaction mixture was cooled to 15°C and 2N HC1 was slowly added (until pH ~1). The reaction mixture was then stirred at room temperature for 20 min and the layers were separated. The aqueous layer (containing the product) was washed with ethyl acetate (300 mL x 3). The aqueous layer was cooled to 0°C and adjusted the pH to ~8 using 20% aqueous NaiCCL solution. The product was extracted with 10% methanol in DCM (300 mL x 3). The combined organic layer was washed with water (300 mL), dried over sodium sulfate and filtered. The filtrate was treated with activated charcoal (16 g, 20% w/w with respect to crude input of 80 g), then the reaction mixture was stirred overnight at room temperature and filtered through Celite ® bed. The bed was washed with 5% methanol in DCM (~ 20 vol, until absence of product by TLC). The filtrate was concentrated under vacuum at 35°C – 40°C to afford compound of formula (I) (70g, HPLC purity: 92.70%, retention time: 15.65 min).
Work-up for improved chiral purity: The above compound of formula (I) was dissolved in ethylacetate (~30 vol, 2L) and washed with aqueous citric acid (2 times, 400 mL x 1 and 200mL x 1), aqueous NaHCCL solution (2%, 500 mL x 1) and aqueous NaCl solution (10%, 500 mL x 1). The combined organic layer was dried over sodium sulfate and filtered. The filtrate was concentrated under vacuum at 35°C – 40°C to afford compound of formula (I) (~60g).
*H NMR (400MHz, DMSO-rfe): d: 10.79 (s, 1H), 10.46 (s, 1H), 8.61 (d, 1H), 8.28 (d, 1H), 8.07-8.05 (m, 1H), 7.69 (s, 1H), 7.56 (d, 1H), 7.39 (m, 2H), 6.84-6.77 (m, 1H), 6.62 (s, 2H), 6.51 (d, 1H), 6.13 (s, 1H) , 3.62-3.59 (m, 4H), 3.35 (d, 1H), 3.15-3.13 (m, 2H), 2.42-2.39 (m, 5H), 1.80-1.77 (m, 1H), 0.98 (d, 3H) , 0.88-0.85 (m, 2H), 0.67 (d, 3H), 0.62-0.60 (m, 2H); LCMS: m/z= 529.25-free base (M+H) + , HPLC: 98.98%, retention time: 15.40 min.
Patent
PATENT
In some embodiments, the compound of formula (I) is (E)-N-(5-(3-(l-((5-cyclopropyl-lH-pyrazol-3-yl)amino)-3-methyl-l-oxobutan-2-yl)phenyl)pyridin-2-yl)-4-morpholinobut-2-enamide or a pharmaceutically acceptable salt or a stereoisomer thereof (Compound 44).
Compound 44 is disclosed in WO 2016/193939 Al, published December 8, 2016, entitled “Substituted heterocyclyl derivatives as cdk inhibitors,” the entire contents of which are incorporated herein by reference. Compound 44A can be in the form of a fumaric acid salt or cocrystal as described in WO 2022/130304 Al, published June 23, 2022, entitled “Cocrystal of a cdk inhibitor,” the entire contents of which are incorporated herein by reference.
Example 3: Synthesis of Compounds 44A & 44B via Chiral Separation
Scheme-1


Step-1: Synthesis of 2-(3-bromophenyl)-3-methylbutanoic acid
[0352] 2M LDA (698 mL, 1.38mol) was added to a solution of 2-(3 -bromophenyl) acetic acid (reagent-1, 150g, 0.69mol) in THF (700mL) at -78 °C over a period of 30 min. The reaction mass was stirred for 2h at -78 °C followed by the drop wise addition of Isopropyl bromide (255 g, 2.07mol) over a period of 30 min at -78 °C. The reaction mass was stirred at room temperature for overnight. The reaction mass was quenched with IN HC1 (pH 2) and product extracted to ethyl acetate (500mL x 3). The combined organic layer washed with water followed by brine, dried and concentrated under reduced pressure to afford the title crude compound which was purified by silica column by eluting with 0-10% ethyl acetate -hexane system to afford the title compound 2 (150g, 83% yield). LCMS: m/z = 254.80 (M-2H)’
Step-2: Synthesis of tert-butyl 3-(2-(3-bromophenyl)-3-methylbutanamido)-5-cyclopropyl-lH-pyrazole-1 -carboxylate
[0353] 2-(3-bromophenyl)-3-methylbutanoic acid (intermediate-2, 70g, 0.0.27mol) was dissolved in dry DCM (500 mL) and added oxalyl chloride (68 mL, 0.78mol) dropwise at 0 °C followed by addition of catalytic amount of DMF (0.8mL) and maintained reaction mass at same temperature for 30min. The reaction mass was allowed to room temperature and stirred for 4h, distilled off the solvent and excess oxalyl chloride under vacuum. Re-dissolved the residue in DCM (250 mL) and added slowly to the cooled solution of tert-butyl 3 -amino-5 -cyclopropyl- 1H-pyrazole-1 -carboxylate (intermediate-3, 49g, 0.218mol) and TEA (55 mL, 0.546mol) in THF (250 mL) at 0 °C for 30min, The reaction was stirred at room temperature for 12h then the reaction mass was concentrated under reduced pressure and the residue was dissolved in DCM, washed with saturated NaHCO3 solution and brine. The organic layer was dried over anhydrous sodium sulphate and concentrated under reduced pressure, the crude was purified by silica gel column chromatography by eluting with 15% ethyl acetate-hexane to afford the title compound 4 (90g, 71% ) LCMS: m/z = 363.80 (M-Boc+2).
Step-3: Synthesis of tert-butyl 5-cyclopropyl-3-(3-methyl-2-(3-(4,4,5,5-tetramethyl-l,3,2-dioxaborolan-2-yl)phenyl)butanamido)-l H -pyr azole- 1 -carboxylate
[0354] To a degassed solution of tert-butyl 3-(2-(3-bromophenyl)-3-methylbutanamido)-5-cyclopropyl-lH-pyrazole-1 -carboxylate (intermediate-4, 90g, 0.193mol) and 4, 4, 4′, 4′, 5, 5, 5′, 5′-octamethyl-2,2′-bi(l,3,2-dioxaborolane) (62g, 0.25 Imol) in 1,4-Dioxane (500 mL) was added potassium acetate (37.80g, 0.386mol). The reaction mass was allowed to stir for 10 min with degassing at RT and added PdC12(dppf).DCM complex (12.5g, 0.015mol). The reaction mass was heated for 3-4 h at 100 °C. Reaction mixture cooled to RT and filtered on celite bed, filtrate evaporated to get dark brown liquid. The crude material was purified by silica column chromatography by eluting with 20% ethyl acetate in hexane to afford the compound 5 (90g, 86%). LCMS: m/z = 410 (M-Boc+1)+.
Step-4: Synthesis of (E)-N-(5-(3-(l-((5-cyclopropyl-lH-pyrazol-3-yl)amino)-3-methyl-l-oxobutan-2-yl)phenyl)pyridin-2-yl)-4-morpholinobut-2-enamide
[0355] To a degassed solution of tert-butyl 5-cyclopropyl-3-(3-methyl-2-(3-(4, 4,5,5-tetramethyl- 1 ,3 ,2-dioxaborolan-2-yl)phenyl)butanamido)- 1 H-pyrazole- 1 -carboxylate, 5 (10g, 0.019mol) and (E)-N-(5-bromopyridin-2-yl)-4-morpholinobut-2-enamide (7.7g, 0.023mol) in
1,4-Dioxane (lOOmL) and water (40mL) followed by Cs2CO3 (14.5g, 0.045mol) were added. The reaction mass was allowed to stir for 10 min with degassing and added Pd(PPh3)4 (1.1g, 0.00095mol), heated the reaction mass for 4 h at 100 °C in a sealed tube. The reaction mass was cooled and diluted with brine solution. The aqueous layer was separated and re-extracted with ethyl acetate. The combined organic layer was evaporated to dryness and crude material was purified by silica column chromatography by eluting with 10%-l 5 % methanol in DCM to get desired pure compound 44 (4.5g, 44%). LCMS: m/z = 529.15 (M+H)+; HPLC: 95.17%, rt: 6.34 min.
[0356] Racemic (E)-N-(5 -(3 -( 1 -((5 -cyclopropyl- 1 H-pyrazol-3 -yl)amino)-3 -methyl- 1 -oxobutan-2-yl)phenyl)pyridin-2-yl)-4-morpholinobut-2-enamide was separated by using chiral preparative HPLC column (Method: Column: Chiral Pak IA (20mm X 250 mm, 5 micron), Elution: isocratic (50:50), A=ACN, B= MeOH, Flow: 20mL/min ) to afford the pure Isomer- 1 and Isomer-2.
Isomer-1 (Compound 44-A):
[0357] 1HNMR (DMSO-d6, 400MHz): 5 12.02 (s, 1H), 10.78 (s, 1H), 10.44 (s, 1H), 8.61 (s, 1H), 8.28 (d, 1H), 8.07-8.05 (m, 1H), 7.68 (s, 1H), 7.57 (d, 1H), 7.41-7.37 (m, 2H), 6.81-6.78 (m, 1H), 6.49 (d, 1H), 6.13 (s, 1H), 3.61-3.58 (m, 4H), 3.36-3.34 (m, 1H), 3.12 (d, 2H), 2.41-2.32 (m, 5H), 1.82-1.76 (m, 1H), 0.97 (d, 3H), 0.88-0.85 (m, 2H), 0.67 (d, 3H), 0.62-0.59 (m, 2H); LCMS: m/z = 529.15 (M+H)+; HPLC: 96.72%, rt: 6.39 min; Chiral HPLC: 97.68%, rt: 14.47.
Isomer-2 (Compound 44B):
[0358] 1HNMR (DMSO-d6, 400MHz): 5 12.02 (s, 1H), 10.78 (s, 1H), 10.44 (s, 1H), 8.61 (s, 1H), 8.28 (d, 1H), 8.07-8.04 (m, 1H), 7.68 (s, 1H), 7.57 (d, 1H), 7.41-7.37 (m, 2H), 6.81-6.78 (m, 1H), 6.50 (d, 1H), 6.14 (s, 1H), 3.61-3.58 (m, 4H), 3.36-3.34 (m, 1H), 3.12 (d, 2H), 2.40-2.39 (m, 5H), 1.82-1.76 (m, 1H), 0.97 (d, 3H), 0.88-0.85 (m, 2H), 0.67 (d, 3H), 0.62-0.60 (m, 2H); LCMS: m/z = 529.15 (M+H)+; HPLC: 96.24%, rt: 6.39 min; Chiral HPLC: 97.92%, rt: 8.80.
Example 4: Preparation of Compound 44-A via Chiral Synthesis
Preparation of KRM-A (chemical precursor to Compound 44-A)


Step-4
KRM-A
Step-1: Preparation of 2-(3-bromophenyl)-3-methylbutanoic acid (1)
[0359] 2M LDA (698 mL, 1.38mol) was added to a solution of 2-(3 -bromophenyl) acetic acid (150 g, 0.69 mol) in THF (700mL) at -78 °C over a period of 30 min. The reaction mixture was stirred for 2h at -78 °C followed by drop wise addition of isopropyl bromide (XB, 255 g, 2.07 mol) over a period of 30 min at -78 °C. The reaction mass was stirred at room temperature overnight. The reaction mass was quenched with IN HC1 (pH 2) and the obtained product was extracted to ethyl acetate (500 mL x 3). The combined organic layer was washed with water followed by brine, dried over anhydrous sodium sulfate and concentrated under reduced pressure to afford the title crude compound which was purified by silica column by eluting with 0-10% ethyl acetate -hexane system to afford the title compound (150 g, 83% yield), HPLC purity-96%. The compound of formula (1) can also be prepared by the procedure described in CN110590747.
Step-2: Preparation of Compound 3
[0360] 2-(3-bromophenyl)-3-methylbutanoic acid (1, 510 g, 1.98 mol) was dissolved in 30% of IPA in water (10.2 L; 3.06 L of IPA-7.14 L of water) and (1R, 27?)-cyclohexane-l,2-diamine (2, 113 g, 0.9 mol) was added. The reaction mixture was stirred at room temperature for 10 min until the precipitation was observed, then heated to 100 °C till the solution becomes clear and was stirred at same temperature for another 30 min. The reaction mixture was allowed to attain room temperature slowly for 8-12h. The obtained solid was filtered and washed with 500 mL of 30% IPA-water mixture and dried under vacuum to afford the compound 3 (620 g, wet).
[0361] Work up for analysis (for Chiral purity): Small portion (100 mg) of compound 3 was taken in DCM (2-3 mL) and was added IN HC1 (pH 2) at 0 °C till the clear solution was observed. The compound was extracted into DCM, dried over Na2SC>4 and the solvent was evaporated to afford the title compound as white solid (20 mg). Chiral HPLC was recorded for this sample and 20.6% of undesired isomer was observed in chiral HPLC.
[0362] In order to improve the chiral purity of the title compound, the recrystallization method was performed as described below.
Step-3: Recrystallization
[0363] The compound 3 (619.90 g) was taken in 30% of IPA in water (12.4 L), then the mixture was heated to 100 °C till the solution becomes clear and stirred at same temperature for another 30min. The reaction mixture was allowed to attain room temperature slowly for 8-12h. The obtained solid was filtered and washed with 500mL 30% IP A- water and dried under vacuum to afford a desired compound (360g, wet).
[0364] Work up for analysis (for Chiral purity): Small portion (100 mg) from above compound was taken in DCM (2-3mL), was added IN HC1 (pH 2) at 0 °C till the clear solution was observed and the compound was extracted to DCM, dried over Na2SCL and the solvent was evaporated to afford title compound as white solid (35 mg). Chiral HPLC was recorded for this sample and 10.3% of undesired isomer was observed in chiral HPLC.
[0365] The recrystallization method was repeated for three more times by using 30% of IPA in water as described above to get the purity >98.50% ee along with 0.27% other isomer to afford 286 g of compound 4.
Step-4: Preparation of (S)-2-(3-bromophenyl)-3-methylbutanoic acid (KRM-A)
[0366] The compound 4 (286 g) was taken in DCM (1.3 L), then was added IN HC1 at 0 °C until the clear solution was observed, and the compound was extracted to DCM (500 mL x 2). The organic layer was separated and washed brine solution (500 mL) and dried over Na2SO4, the solvent was evaporated to afford title compound as white solid (148 g, 60% yield). Chiral HPLC: 98.50%
[0367] ‘H NMR (400MHz, DMSO-d6): 8 12.5 (s, 1H), 7.50-7.44 (m, 2H), 7.34-7.26 (m, 2H), 3.16 (d, 1H), 2.23-2.11 (m, 1H), 0.98 (d, 3H), 0.63 (d, 3H); Chiral HPLC: 98.50% retention time: 4.588 min.
Preparation of Compound 44-A


Step-1: Synthesis of (S)-2-(3-bromophenyl)-N-(5-cyclopropyl-lH-pyrazol-3-yl)-3-methylhutanamide
Step- la: Preparation of KRM-D
[0368] To a stirred solution of KRM-A (100g, 0.388mol) in dry DCM (600 mL, 6 vol), a catalytic amount of DMF (10 mL) was added followed by oxalyl chloride (45 mL, 0.525 mol) dropwise at 0 °C over a period of 30 min. After completion of addition, the reaction mixture was stirred for 15 min at the same temperature. The reaction mixture was allowed to reach room temperature and stirred for 2 to 4h. After completion of the reaction (reaction was monitored by TLC, acid chloride formation was checked by quenching an aliquot of reaction mixture with MeOH), the reaction mixture was concentrated under vacuum at 40°C-45°C to afford crude 2-(3-bromophenyl)-3 -methylbutanoyl chloride (KRM-D). The crude KRM-D was dissolved in toluene (500mL) and used for next step.
Step- lb: Preparation of compound of formula Z
[0369] (S)-2-(3-bromophenyl)-3 -methylbutanoyl chloride in toluene was added slowly to a pre-cooled solution (0 to 5 °C) of te/7-butyl 3 -amino-5 -cyclopropyl- IH-pyrazole-l -carboxylate (KRM-B, 95.5g, 0.427 mol) and N, N-diisopropylethyl amine (100 mL, 0.583 mol) in toluene (1.2 L) at 0 °C for the period of l-2h. The reaction mixture was allowed to attain RT and stirred for overnight. The reaction mixture was then cooled to 0-5°C and washed with ice-cold 1.5N HCI (3 x 500 mL). The organic layer was washed with sodium bicarbonate solution (500 mL),
brine solution (500 mL), dried over anhydrous Na2SO4, filtered and concentrated under vacuum at 45-50°C to afford crude tert-butyl (5)-5-(2-(3-bromophenyl)-3-methylbutanamido)-3-cyclopropyl-lH-pyrazole-1 -carboxylate (compound of formula Z) as light brown oil (~180g, LCMS: m/z= 461.9 (M+H)+, HPLC: 80.80%, retention time: 15.89 min). The crude product was taken as such for next step without further purification.
Step-lc: Preparation of compound of formula Y
[0370] To a suspension of tert-butyl (S)-5-(2-(3-bromophenyl)-3-methylbutanamido)-3-cyclopropyl-lH-pyrazole-1 -carboxylate (180 g, 1.731 mol) in dioxane (360 mL) was added 2N aqueous HC1 (360 mL) at 0 °C. The reaction mixture was stirred overnight at room temperature.
[0371] After completion of the reaction, dioxane was concentrated, and the reaction mixture was diluted with water (500 mL) and basified with solid sodium bicarbonate (until pH-8). The resulted compound was extracted with DCM (700 mL x 3). The combined organic layers were washed with water (300 mL) and brine solution (300 mL), and dried over anhydrous Na2SO4. The organic layer was concentrated to get a crude (<S)-2-(3-bromophenyl)-N-(5-cyclopropyl-lH-pyrazol-3-yl)-3-methylbutanamide (Compound of formula Y) as a semi solid. The crude was dissolved in toluene (500 mL) and the solution was stirred for 18 h. The solid formed was filtered and washed with toluene (100 mL) and n-heptane (200 mL). The solid was further dried under vacuum at 45-50°C for 6 h to afford a title compound (110g, Yield: 78% over two steps). LCMS: m/z= 362 (M+H)+, HPLC: 97.66%, retention time: 24.10 min
[0372] Step-2: Preparation of (S, E)-N-(5-(3-(l-((5-cyclopropyl-lH-pyrazol-3-yl) amino)-3-methyl-l-oxobutan-2-yl) phenyl) pyridin-2-yl)-4-morpholinobut-2-enamide (Compound 44A)
[0373] To a degassed solution of (<S)-2-(3-bromophenyl)-N-(5-cyclopropyl-lH-pyrazol-3-yl)-3-methylbutanamide (50 g, 0.138 mol) and (£)-4-morpholino-N-(5-(4,4,5,5-tetramethyl-l,3,2-dioxaborolan-2-yl)pyridin-2-yl)but-2-enamide (KRM-C, 56.6 g, 0.151 mol, 1.1 eq) (prepared according to the procedure described in W02020202001) in 1,4-dioxane (500 mL, 10 vol) and water (100 mL, 2 vol) was added K3PO4 tribasic (73.2 g, 0.345 mol, 2.5 eq) at room temperature The reaction mass was stirred for 20 min with argon purging (degassing). Pd(dppf)C12.DCM [l,l’-Bis(diphenylphosphino)ferrocene]dichloropalladium(II) complex with dichloromethane] (3.38 g, 0.0042 mol, and 0.03eq) was added and the reaction mixture was heated to 90°C for 1-2 h (The reaction was monitored by TLC using 10% methanol in DCM as solvent system).
[0374] After completion of the reaction, the reaction mass was cooled to room temperature and filtered through Celite® bed. The bed was washed with 1, 4-dioxane (200 mL) and the filtrate was concentrated to get crude compound. The crude compound was dissolved in 5% methanol in DCM (400 mL) and washed with water (200 mL x 2). The aqueous layer was separated and extracted with DCM (100 mL x 2). The combined organic layer was washed with brine solution, filtered and dried over sodium sulphate. The organic layer was concentrated under vacuum at 35-40°C to get crude title compound (~80g).
[0375] The crude compound 44A, (80 g) was dissolved in 700 mL of ethyl acetate. The reaction mixture was cooled to 15°C and 2N HC1 was slowly added (until pH ~1). The reaction mixture was then stirred at room temperature for 20 min and the layers were separated. The aqueous layer (containing the product) was washed with ethyl acetate (300 mL x 3). The aqueous layer was cooled to 0°C and adjusted the pH to ~8 using 20 % aqueous Na2COs solution. The product was extracted with 10% methanol in DCM (300 mL x 3). The combined organic layer was washed with water (300 mL), dried over sodium sulphate and filtered. The filtrate was treated with activated charcoal (16 g, 20% w/w with respect to crude input of 80 g), stirred overnight at room temperature and filtered through Celite® bed. The bed was washed with 5% methanol in DCM (~ 20 vol, till absence of product by TLC). The filtrate was concentrated under vacuum at 35°C – 40°C to afford compound 44A (70g, HPLC purity: 92.70%, retention time: 15.65 min).
[0376] ‘ H NMR (400MHz, DMSO-^): <5: 10.79 (s, 1H), 10.46 (s, 1H), 8.61 (d, 1H), 8.28 (d, 1H), 8.07-8.05 (m, 1H), 7.69 (s, 1H), 7.56 (d, 1H), 7.39 (m, 2H), 6.84-6.77 (m, 1H), 6.62 (s, 2H), 6.51 (d, 1H), 6.13 (s, 1H), 3.62-3.59 (m, 4H), 3.35 (d, 1H), 3.15-3.13 (m, 2H), 2.42-2.39 (m, 5H), 1.80-1.77 (m, 1H), 0.98 (d, 3H), 0.88-0.85 (m, 2H), 0.67 (d, 3H), 0.62-0.60 (m, 2H);
LCMS: m/z= 529.25-free base (M+H)+, HPLC: 98.98%, retention time: 15.40 min.


AS ON DEC2021 3,491,869 VIEWS ON BLOG WORLDREACH AVAILABLEFOR YOUR ADVERTISEMENT

join me on Linkedin
Anthony Melvin Crasto Ph.D – India | LinkedIn
join me on Researchgate
RESEARCHGATE
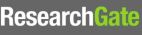
join me on Facebook
Anthony Melvin Crasto Dr. | Facebook
join me on twitter
Anthony Melvin Crasto Dr. | twitter
+919321316780 call whatsaapp
EMAIL. amcrasto@gmail.com
//////////
REF
POSTER SESSION: Molecular Targeted Agents| Volume 138, SUPPLEMENT 2, S47, October 01, 2020
//////Tacaciclib, GTPL12880, AUR-102
CC(C)C(C1=CC=CC(=C1)C2=CN=C(C=C2)NC(=O)C=CCN3CCOCC3)C(=O)NC4=NNC(=C4)C5CC5

NEW DRUG APPROVALS
ONE TIME
$10.00
CAREBASTINE
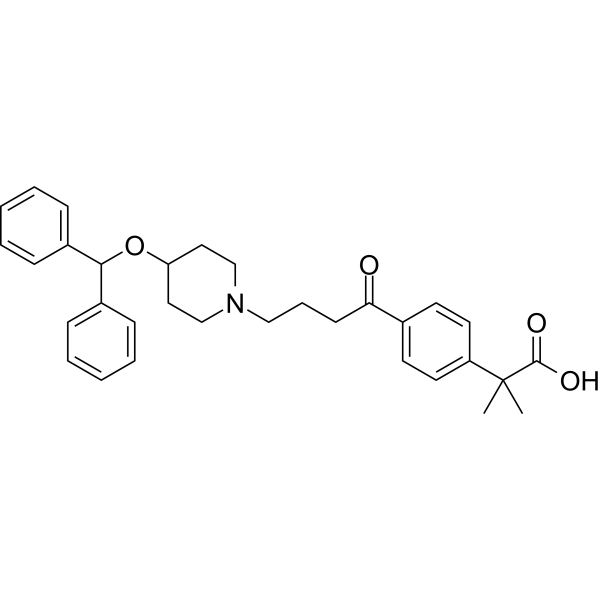
CAREBASTINE
Molecular Weight | 499.64 |
---|---|
Appearance | Solid |
Formula | C32H37NO4 |
CAS No. | 90729-42-3 |
Carebastine is the active metabolite of Ebastine. Carebastine is a histamine H1 receptor antagonist. Carebastine inhibits VEGF-induced HUVEC and HPAEC proliferation, migration and angiogenesis in a dose-dependent manner. Carebastine suppresses the expression of macrophage migration inhibitory factor.
Carebastine is the active metabolite of Ebastine. Carebastine is a histamine H1 receptor antagonist. Carebastine inhibits VEGF-induced HUVEC and HPAEC proliferation, migration and angiogenesis in a dose-dependent manner[1]. Carebastine suppresses the expression of macrophage migration inhibitory factor[2].
Literature References: Nonsedating type histamine H1-receptor antagonist. Prepn: J. M. P. Soto et al., EP 134124; eidem, US 4550116 (both 1985 to Fordonal). Metabolized in vivo to carebastine, its active carboxylic acid metabolite.

PATENT
https://patents.google.com/patent/US8067604B2/en
These schemes also illustrate the interrelatedness of the processes and intermediates.










EXAMPLE 1
One gram of 9 was dissolved in 20 mL of DMF and 18 mg of P(tBu)3, 41 mg of Pd(dba)2, 230 mg of ZnF2 and 1.2 g of 5 were added. A mixture was stirred at 80° for 18 hours, cooled to room temperature, diluted with ether and washed with water. The organic layer was dried over sodium sulfate, filtered and stripped in vacuo. The resulting product was flash chromatographed on silica gel using 4:1 hexane ethyl acetate to yield 1.0 g (91%) of 10. A repeat of the reaction on larger scale using 15 g of 9 provided 15.2 g (93%) of 10.
EXAMPLE 2
Five grams of 9 was dissolved in 50 mL of methylene chloride and cooled to 0° C. To the solution was added 5.78 g of trimethylsilyl iodide. The mixture was stirred for 30 minutes and excess sodium bisulfite solution was added with vigorous stirring at room temperature. The layers were separated and the aqueous layer extracted twice with methylene chloride. Combined organic layers were dried, filtered and stripped in vacuo to provide 7.7 g (98%) of 1. The reaction was repeated on a larger scale using 15 g of 9 to produce 22.5 g of 1 (96%) yield.
EXAMPLE 3
Six grams of potassium carbonate, 5.8 g of piperidine 2 and 7.6 g of 1 are combined in 100 mL of DMF. The suspension is stirred at room temperature until TLC in 4:1 hexane-ethyl acetate indicates a complete reaction. The reaction mixture is poured into 400 mL of water and extracted three times with methylene chloride. The combined organic extracts are dried, filtered and reduced in vacuo. The resulting product is flash chromatographed on silica gel using ethyl acetate containing 10% triethylamine to yield 3.
EXAMPLE 4
Seven grams of 3 is dissolved in 100 mL of methanol, cooled to 0° C. and 1.1 g of sodium borohydride is added. The mixture is stirred 1 hour, concentrated and partitioned between ethyl acetate and saturated aqueous sodium bicarbonate. The bicarbonate layer is extracted twice with ethyl acetate, the combined organic layers are dried over sodium sulfate and the solution is reduced in vacuo to provide 4.
EXAMPLE 5
Two grams of 4 is dissolved in 30 mL of DMF. To this are added 16.2 mg of P(tBu)3, 36.6 mg of Pd(dba)2, 209 mg of ZnF2 and 1.056 g of 5. The mixture is heated at 80° C., cooled, diluted with ether and worked up as in example 1. The resulting product is flash chromatographed on silica gel using 9:1 ethyl acetate-triethylamine to provide 7.
EXAMPLE 6
One hundred fifty milligrams of 6 is slurried in 5 mL of water and 10 mL of methanol. To the slurry is added 175 mg of sodium hydroxide. The slurry is refluxed for one hour, cooled to room temperature and the methanol removed in vacuo. The resulting aqueous solution is distributed between water and chloroform, the chloroform layer is discarded, the aqueous layer is adjusted to pH 2.3 and extracted with chloroform. The organic layer is dried, filtered and reduced in vacuo to provide carebastine.
EXAMPLE 7
Five grams of 1 was combined with 2.64 g of 2 and 2.0 g of potassium carbonate and 80 mL of DMF. The mixture was stirred at room temperature for two hours, poured into 400 mL of water and extracted three times into methylene chloride. The combined organic layers were dried, filtered and reduced in vacuo. The resulting product was flash chromatographed on silica gel using 9:1 ethyl acetate-triethylamine to provide 2.0 g (54%) of 3.
EXAMPLE 8
One and seven-tenths grams of 3, 90 mg of P(tBu)3, 300 mg of Pd(dba)2, 250 mg of ZnF2 and 1.1 g of 5 were dissolved in 330 mL of DMF under argon. The mixture was heated to 80° for two hours, cooled to room temperature, diluted with ether and worked up as described in example 1. The resulting product was filtered through silica to provide 1.2 g (67.8%) of 6.
EXAMPLE 9
Two grams of 20, 170 mg of P(tBu)3, 560 mg of Pd(acac)2, 474 mg of ZnF2 and 2.0 g of 5 were combined in 50 mL of DMF under argon. The mixture was heated to 80° C. and monitored by HPLC. When reaction was complete, the mixture was cooled to room temperature and 250 mL of water was added. The mixture was extracted three times with ether, dried, filtered and reduced in vacuo. The resulting product was flash chromatographed in 4:1 hexane-ethyl acetate to provide 1.89 g (85%) of 8.
EXAMPLE 10
Two grams of the triflate analog of 20 were reacted as in the foregoing example with 134 mg P(tBu)3, 433 mg of Pd(acac)2, 375 mg of ZnF2 and 1.58 g of 5 to provide 1.56 g (90% yield) of 8.
Example 11
Piperidinol 25 is reacted with chlorodiphenylmethane as described in Fujii et al. Arzneim.-Forsch. 44, 527-538 (1994) to provide 6.
PATENT
WO/2023/213182CAREBASTINE SALT AND USE THEREOF
WIPO – Search International and National Patent Collections
Example 1: Potassium 2-(4-(4-(4-(diphenylmethoxy)piperidin-1-yl)butyryl)phenyl)-2-methylpropionate (carristin potassium salt ) preparation
[0060]
[0061]
Step 1: Preparation of methyl 2-(4-(4-(4-(diphenylmethoxy)piperidin-1-yl)butyryl)phenyl)-2-methylpropionate
[0062]
[0063]
Add 4-(diphenylmethoxy)piperidine hydrochloride (473mg, 1.77mmol), DMAC (4.5ml), K 3 PO 4 (1.13g, 5.3mmol), KI (29mg, 0.177mmol) to a 25ml single-neck bottle. , stir and heat to 100°C. Weigh 2-[4-(4-chloro-1-butyryl)phenyl]-2-methylpropionate methyl ester (600mg, 2.12mmol) and dissolve it in 1ml of DMAC. Add the reaction solution slowly and dropwise, and keep the reaction for 4~ 6h, TLC detects that the raw material reaction is complete. Cool to room temperature, add isopropyl acetate and water, and stir to separate layers. The aqueous phase was then extracted with isopropyl acetate, the organic phases were combined, washed twice with water, dried over anhydrous sodium sulfate, filtered, concentrated, and passed through a silica gel column to obtain 500 mg of the title product, yield 45%, purity: 97.3%.
[0064]
ESI-MS: m/z = 514.3(M+H) +。
[0065]
1H NMR (400 MHz, CDCl 3) δ: 7.93 (d, J=8.3Hz, 2H), 7.47 (m, 4H), 7.42 (d, J=8.3Hz, 2H), 7.30 (m, 4H), 7.18 (m, 2H), 3.64 (s, 3H),2.98 (m, 4H), 2.42 – 2.40 (m, 4H), 1.96 (m, 4H), 1.62 (s, 6H), 1.42 (m, 4H)。
[0066]
Step 2: Preparation of 2-(4-(4-(4-(Diphenylmethoxy)piperidin-1-yl)butyryl)phenyl)-2-methylpropionic acid (carristin)
[0067]
[0068]
Add (5-methyl-2-oxo-1,3-dioxo-4-yl)methyl-2-(4-(4-(4-(diphenylmethoxy))piperidine-1 to a 25ml three-necked flask) -Methyl)-butyryl)phenyl)-2-methylpropionate (320 mg, 0.62 mmol), 1.5 ml of methanol, 2 ml of 10% NaOH, heated to 60°C for 2 hours, and the TLC raw material reaction was completed. After the reaction is completed, cool to room temperature, concentrate to dryness, add EA, add hydrochloric acid to adjust the pH to 2~3, layer the layers, wash once with water, dry the organic phase, and concentrate to dryness to obtain 300 mg of the title product. Yield: 95%, purity 95.0%.
[0069]
ESI-MS: m/z = 500.3(M+H) +。
[0070]
1H NMR (400 MHz, CDCl 3) δ:7.75-7.63 (m, 2H), 7.57–7.24 (m,12H), 5.48 (s,1H),3.73 (m, 1H), 3.05–3.02 (m, 2H), 2.77–2.66 (m, 6H), 2.20–2.07 (m, 2H), 2.00–1.81 (m,4H), 1.58 (s, 6H)。
[0071]
Step 3: Potassium 2-(4-(4-(4-(Diphenylmethoxy)piperidin-1-yl)butyryl)phenyl)-2-methylpropionate (Carristine Potassium Salt) Preparation
[0072]
[0073]
Add 2-(4-(4-(4-(diphenylmethoxy)piperidin-1-yl)butyryl)phenyl)-2-methylpropionic acid (499mg, 1mmol) and acetonitrile 3.5 to a 25ml three-necked flask. ml, heated to 60°C, added potassium hydroxide (56 mg, 1 mmol), stirred, cooled down, a white solid precipitated, filtered, and dried to obtain 500 mg of carristine potassium salt, with a yield of 90% and a purity of 98.67%.
[0074]
ESI-MS: m/z = 500.3(M+H) +。
[0075]
1H NMR (400 MHz, CDCl 3) δ:7.75-7.63 (m, 2H), 7.57–7.24 (m,12H), 5.48 (s,1H),3.73 (m, 1H), 3.05–3.02 (m, 2H), 2.77–2.66 (m, 6H), 2.20–2.07 (m, 2H), 2.00–1.81 (m,4H), 1.58 (s, 6H)。
[0076]
Example 2: Sodium 2-(4-(4-(4-(diphenylmethoxy)piperidin-1-yl)butyryl)phenyl)-2-methylpropionate (carristine sodium salt ) preparation
[0077]
[0078]
In this example, the preparation method of 2-(4-(4-(4-(diphenylmethoxy)piperidin-1-yl)butyryl)phenyl)-2-methylpropionic acid is the same as in Example 1.
[0079]
Add 2-(4-(4-(4-(diphenylmethoxy)piperidin-1-yl)butyryl)phenyl)-2-methylpropionic acid (499mg, 1mmol) and acetonitrile 3.5 to a 25ml three-necked flask. ml, heated to 60°C, added sodium hydroxide (40 mg, 1 mmol) and stirred for 1 hour, concentrated to dryness, added methyl tert-butyl ether and stirred, filtered, and dried to obtain 458 mg of carristin sodium salt, yield 85%, purity 96.98 %.
[0080]
ESI-MS: m/z = 500.3(M+H) +。
[0081]
1H NMR (400 MHz, CDCl 3) δ:7.75-7.63 (m, 2H), 7.57–7.24 (m,12H), 5.48 (s,1H),3.73 (m, 1H), 3.05–3.02 (m, 2H), 2.77–2.66 (m, 6H), 2.20–2.07 (m, 2H), 2.00–1.81 (m,4H), 1.58 (s, 6H)。
//////////////


AS ON DEC2021 3,491,869 VIEWS ON BLOG WORLDREACH AVAILABLEFOR YOUR ADVERTISEMENT

join me on Linkedin
Anthony Melvin Crasto Ph.D – India | LinkedIn
join me on Researchgate
RESEARCHGATE
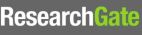
join me on Facebook
Anthony Melvin Crasto Dr. | Facebook
join me on twitter
Anthony Melvin Crasto Dr. | twitter
+919321316780 call whatsaapp
EMAIL. amcrasto@amcrasto
///////////

NEW DRUG APPROVALS
ONE TIME
$10.00